Modelling and Analyzing Inter-Satellite Relative Motion
aerospace
Modelling and Analyzing Inter-Satellite Relative Motion (TOP2-312)
PATENT ONLY. NO SOFTWARE AVAILABLE.
Overview
Swarm conceptsmultiple satellites flying in formation near one another in similar orbitsare of growing interest in the small satellite community. The capabilities needed to support swarm missions go beyond operator-specified geometry, alignment, or separation, but also cross-link communication with maintaining position in the formation. Swarm station-keeping poses a planning challenge due to the limited scalability of ground resources. To address scalable control of orbital dynamics, NASA Ames has patented SODA Swarm Orbital Dynamics Advisor a solution that accepts high-level configuration commands and provides the orbital maneuvers needed to achieve the desired type of swarm relative motion. Rather than conventional path planning, SODAs innovation is the use of artificial potential functions to define boundaries and keep-out regions.
The Technology
Swarms of large numbers of cooperating satellites will introduce new space mission capabilities and complexities. From a mission operations perspective, swarms pose a planning challenge due to the limited scalability of ground operations. The approach of planning and commanding individual satellites simply does not scale for multi-sat swarms of tens or hundreds. If the current state-of-practice continues to be applied, operation of large swarms (e.g., 100 spacecraft or more) will become intractable and cost prohibitive. To avoid this operations bottleneck, a new approach
is required: the swarm must operate as a unit, responding to high level commands and constraints. Swarm Orbital Dynamics Advisor (SODA) enables high level user inputs in a single planning cycle. From one high level command, SODA determines all of the required individual satellite maneuvers over time, relieving ground personnel of the tasks of designing and commanding the placement of the swarm members. SODA provides the orbital maneuvers required to achieve a desired type of relative swarm motion. The purpose of SODA is two-fold. First, it encompasses the algorithms and orbital dynamics model to enable the desired relative motion of the swarm satellites. Second, SODA is compatible with a variety of visualization tools. The purpose of SODAs visualization element is to illustrate this concept clearly with a variety of graphics and animations. After computing the optimal orbital maneuvers to modify the swarm, these results are simulated to demonstrate successful swarm control.
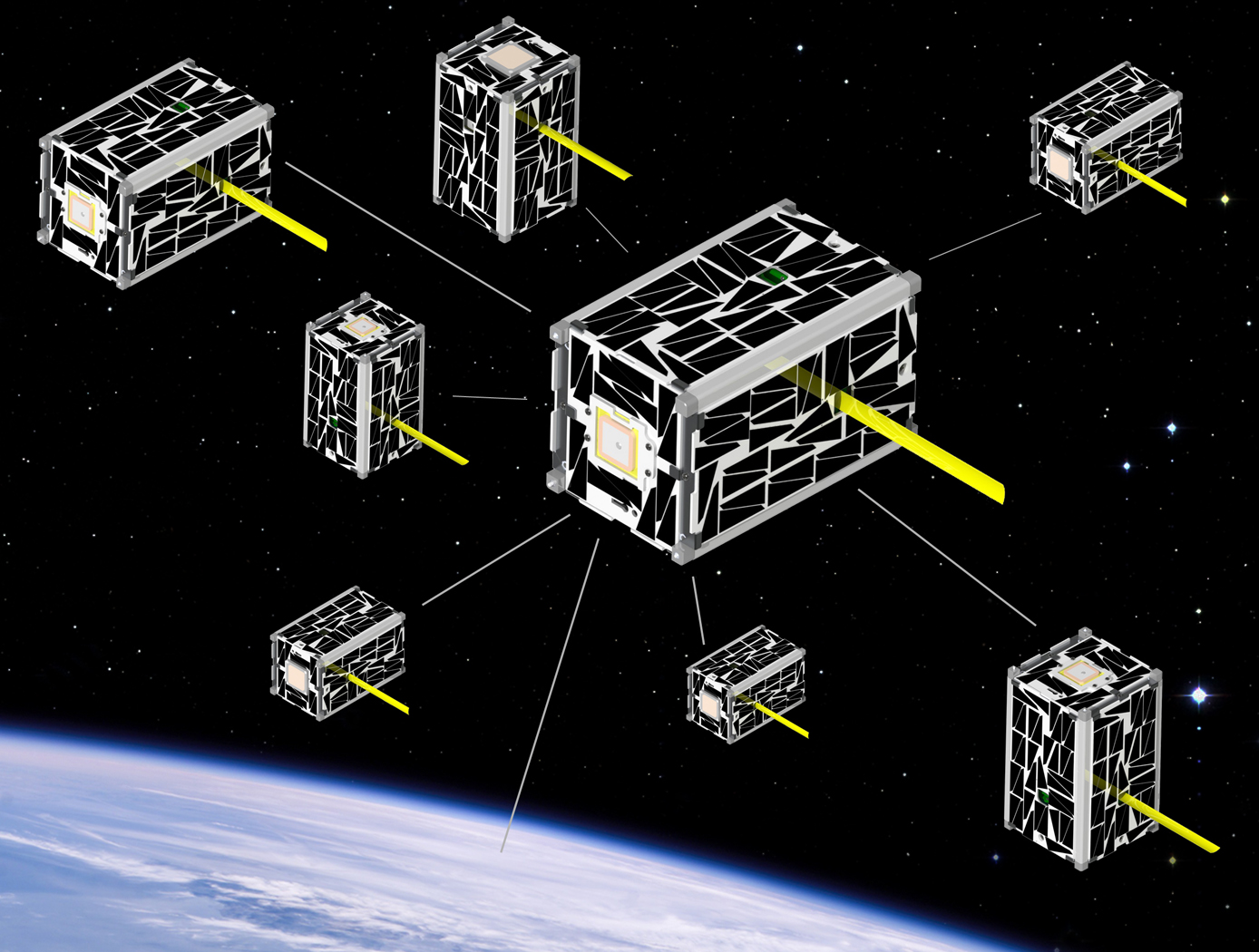
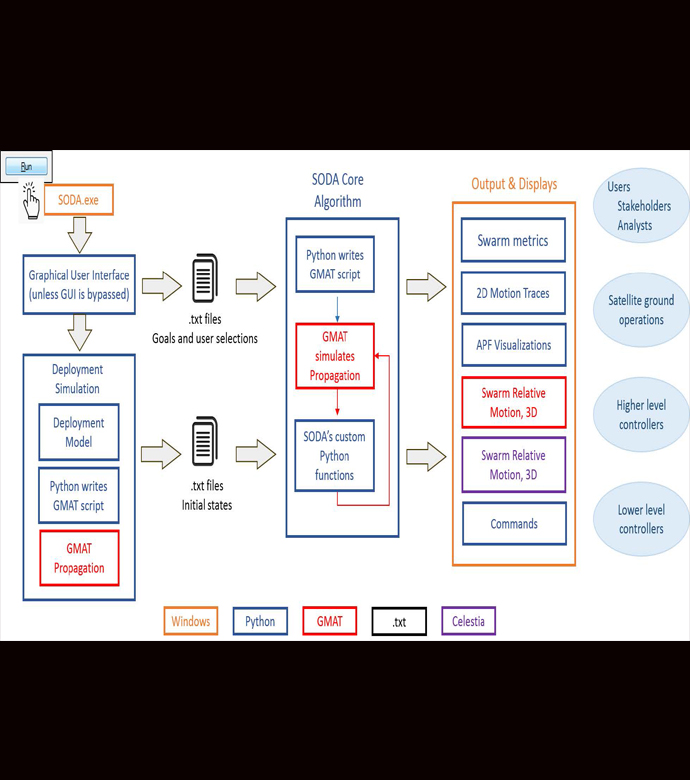
Benefits
- Allows satellite swarms to be controlled by one high level command
- Compatibility with a wide variety of visualization tools
- Useful for multiple swarm types, including in-train distribution and an ellipsoid volume container
- Multiple interfaces for use: graphical user interface for small analyses or developing customer awareness, text files for "hands-off" applications such as Monte Carlo analyses, and a defined software interface for future integration with higher- and lower-level users/controllers
- Swarm deployment and controller design becomes an exercise in simultaneously containing the satellites, thereby decreasing the risk of collision
Applications
- Satellite industry
- Swarm missions
- Interferometric synthetic aperture radar (InSAR) monitoring
- Ground-based laser communications to a chief satellites that can be calibrated with the assistance of atmospheric probe lasers from deputy satellites to reduce power loss
- Rapid stereographic imaging and other sparse antenna array applications
Technology Details
aerospace
TOP2-312
ARC-17997-1
PATENT ONLY. NO SOFTWARE AVAILABLE.
https://www.nasa.gov/feature/swarmsoda/
https://images.nasa.gov/details-ellipsoid_container_swarm_example2.html
https://images.nasa.gov/details-in_train_swarm_example1.html
https://ntrs.nasa.gov/citations/20170011298
https://www.nasa.gov/feature/swarmsoda/
https://images.nasa.gov/details-ellipsoid_container_swarm_example2.html
https://images.nasa.gov/details-in_train_swarm_example1.html
https://ntrs.nasa.gov/citations/20170011298
Similar Results

Dellingr 6U CubeSat
A NASA team gave itself just one year to develop, test and integrate a CubeSat that could reliably and easily accommodate agency-class science investigations and technology demonstrations at a lower cost. The CubeSat known as Dellingr, a name derived from the god of the dawn in Norse mythology will carry three heliophysics-related payloads. It doubles the payload capability of the ubiquitous and proven three-unit, or 3U, CubeSat pioneered by the California Polytechnic Institute in 1999 primarily for the university community.
The need for such a platform, which measures about 12 inches long, nearly 8 inches wide and 4 inches high, was for more cost-effective approaches to achieve compelling Earth and space science.
Disadvantages of the 3U size include more constraints on volume and power. Furthermore, some studies suggest that previous CubeSats failed 40 percent of the time. By doubling the platform's girth, increasing its power capacity, and employing novel processes to increase its on-orbit reliability, the team believes it will have created a platform capable of carrying out more robust missions for science.
Once successfully demonstrated, the team says it will make the platform's design implemented with low-cost, commercial off-the-shelf parts available to any U.S. organization interested in using it.

Algorithms for stabilizing intelligent networks
Some of the current challenges faced by research in artificial intelligence and autonomous control systems include providing self control, resilience, adaptability, and stability for intelligent systems, especially over a long period of time, in changing environments. The Evolvable Neural Software System (ENSS), Formulation for Emotion Embedding in Logic Systems (FEELS), Stability Algorithm for Neural Entities (SANE), and the Logic Expansion for Autonomously Reconfigurable Neural Systems (LEARNS) are foundations for tackling some of these challenges, by providing the basic algorithms evolvable systems could use to manage its own behavior.
These algorithms would allow networks to self regulate, noticing unusual behavior and the circumstances that may have caused that behavior, and then correcting to behave more predictably when similar circumstances are encountered. The process is similar to how psychology in organisms evolved iteratively, eventually finding and keeping better responses to given stimuli.

Monitoring and Control of Each Nanosatellite within a Cluster of Nanosatellites
The key to ElectroMagnets, and Resonant Inductive Coupling (MEMRIC) is the use of low-power electromagnets for relative motion control, a magnetometer for relative distance determination, and a resonant inductive coupling system for power sharing. Traditional satellite buses house all of the major subsystems in one package. Nanosat clustering allows for the distribution of subsystems; each nanosat housing a specific subsystem (functional fractionation). MEMRIC makes this type of clustering possible, allowing a collection of system-specific nanosats to serve as a set of basic functional building blocks. Power collection, communication, navigation, computational, and propulsion units can all be combined to meet various mission requirements, greatly reducing the need for non-recurring design efforts. As new technologies for power, communications, and computation become available, these technologies can be incorporated into standardized nanosat units without the need for redesigning the other units or an entire system. Thus, the capabilities of spacecraft clusters composed of MEMRIC-enabled nanosats can evolve at the pace of technology, without incurring the large costs inherent to redesign of large, complex spacecraft.

Closed Ecological System Network Data Collection, Analysis, Control, and Optimization System
The technology relates generally to controlled ecosystems, and more particularly, to a Controlled Closed-Ecosystem Development System (CCEDS) that can be used to develop designs for sustainable, small-scale reproductions of subsets of the Earths biosphere and the Orbiting Modular Artificial-Gravity Spacecraft (OMAGS). The technology encompassing a CCEDS includes one or more a Closed Ecological Systems (CESs), each having one or more Controlled Ecosystem Modules (CESMs). Each CESM can have a biome containing at least one organism, and equipment comprising one or more of sensors, actuators, or components that are associated with the biome. A controller operates the equipment to effect transfer of material among CESMs to optimize one or more CESM biomes with respect to their organism population health, resilience, variety, quantities, biomass, and sustainability. A CES is a community of organisms and their resources that persist in a sealed volume such that mass is not added or removed. The mass (food/air/water) required by the CES organisms is continually recycled from the mass (waste) produced by the organisms. Energy and information may be transferred to and from a CES. CES research promises to become a significant resource for the resolution of global ecology problems which have thus far been experimentally inaccessible and may very well prove an invaluable resource for predicting the probable ecological consequences of anthropogenic materials on regional ecosystems. In order to create CESs that are orders of magnitude smaller than the Earth that can function without the Earth, the desired gravity level and necessary radiation shielding must be provided by other means. Orbiting Modular Artificial-Gravity Spacecraft (OMAGS) is a fractional gravity spacecraft design for CES payloads and is depicted in Figures below. In tandem, the CCEDS and OMAGS systems can be used to foster gravitational ecosystem research for developing sustainable communities in space and on Earth.

Space Traffic Management (STM) Architecture
As ever larger numbers of spacecraft seek to make use of Earth's limited orbital volume in increasingly dense orbital regimes, greater coordination becomes necessary to ensure these spacecraft are able to operate safely while avoiding physical collisions, radio-frequency interference, and other hazards. While efforts to date have focused on improving Space Situational Awareness (SSA) and enabling operator to operator coordination, there is growing recognition that a broader system for Space Traffic Management (STM) is necessary. The STM architecture forms the framework for an STM ecosystem, which enables the addition of third parties that can identify and fill niches by providing new, useful services. By making the STM functions available as services, the architecture reduces the amount of expertise that must be available internally within a particular organization, thereby reducing the barriers to operating in space and providing participants with the information necessary to behave responsibly. Operational support for collision avoidance, separation, etc., is managed through a decentralized architecture, rather than via a single centralized government-administered system.
The STM system is based on the use of standardized Application Programming Interfaces (API) to allow easier interconnection and conceptual definition of roles to more easily allow suppliers with different capabilities to add value to the ecosystem. The architecture handles basic functions including registration, discovery, authentication of participants, and auditable tracking of data provenance and integrity. The technology is able to integrate data from multiple sources.