Enhanced Fabrication Improves Temperature Sensing in Cryogenic Humid Environments
sensors
Enhanced Fabrication Improves Temperature Sensing in Cryogenic Humid Environments (DRC-TOPS-25)
Useful for energy, industrial, and aerospace applications
Overview
Researchers at NASA's Armstrong Flight Research Center have developed a new manufacturing process that improves the ability of fiber optic sensing systems to measure temperature and liquid levels when operating in humid environments. The process involves eliminating moisture from the optical fiber coating, then completing the sensor assembly within humidity-controlled conditions. The resulting sensor hardware provides precise and accurate measurements even when operating in a humid environment. Originally designed to monitor a rocket's cryogenic fuel levels in conjunction with NASA's patented Fiber Optic Sensing System (FOSS), this technology can be used in many industrial, food, and medical applications.
The Technology
This technology was developed to improve Armstrong's multi-patented FOSS system, which has long been used to measure temperature and liquid levels in cryogenic environments. When the sensing system's fibers trapped humidity from the surrounding environment before their submersion into cryogenic liquids, the moisture adversely affected outputs. A new manufacturing process solves this problem, increasing reliability and accuracy not only of NASA's FOSS but also any fiber optic sensing system.
How It Works
Armstrong has developed a two-step process to assemble the sensors. First, the bare sensor fiber is inserted into an oven to expel all moisture from the fiber coating. Then, the moisture-free fiber is placed inside a humidity-controlled glove box to prevent it from absorbing any new moisture. While inside the glove box, the fiber is inserted into a loose barrier tubing that isolates the fiber yet is still thin enough to provide adequate thermal transfer. The tubing can be further purged with various gases while it is inside the glove box to provide additional moisture isolation.
This innovation is particularly useful for fiber optic systems that measure temperature and that identify any temperature stratifications within cryogenic liquids.
Why It Is Better
This process seals sensor fibers from environmental moisture, enabling fiber optic sensing systems to operate reliably in humid environments. The innovation eliminates erroneous readings that can occur due to moisture collection on the fiber sensors.
For more information about the full portfolio of FOSS technologies, see DRC-TOPS-37 or visit https://technology-afrc.ndc.nasa.gov/featurestory/fiber-optic-sensing
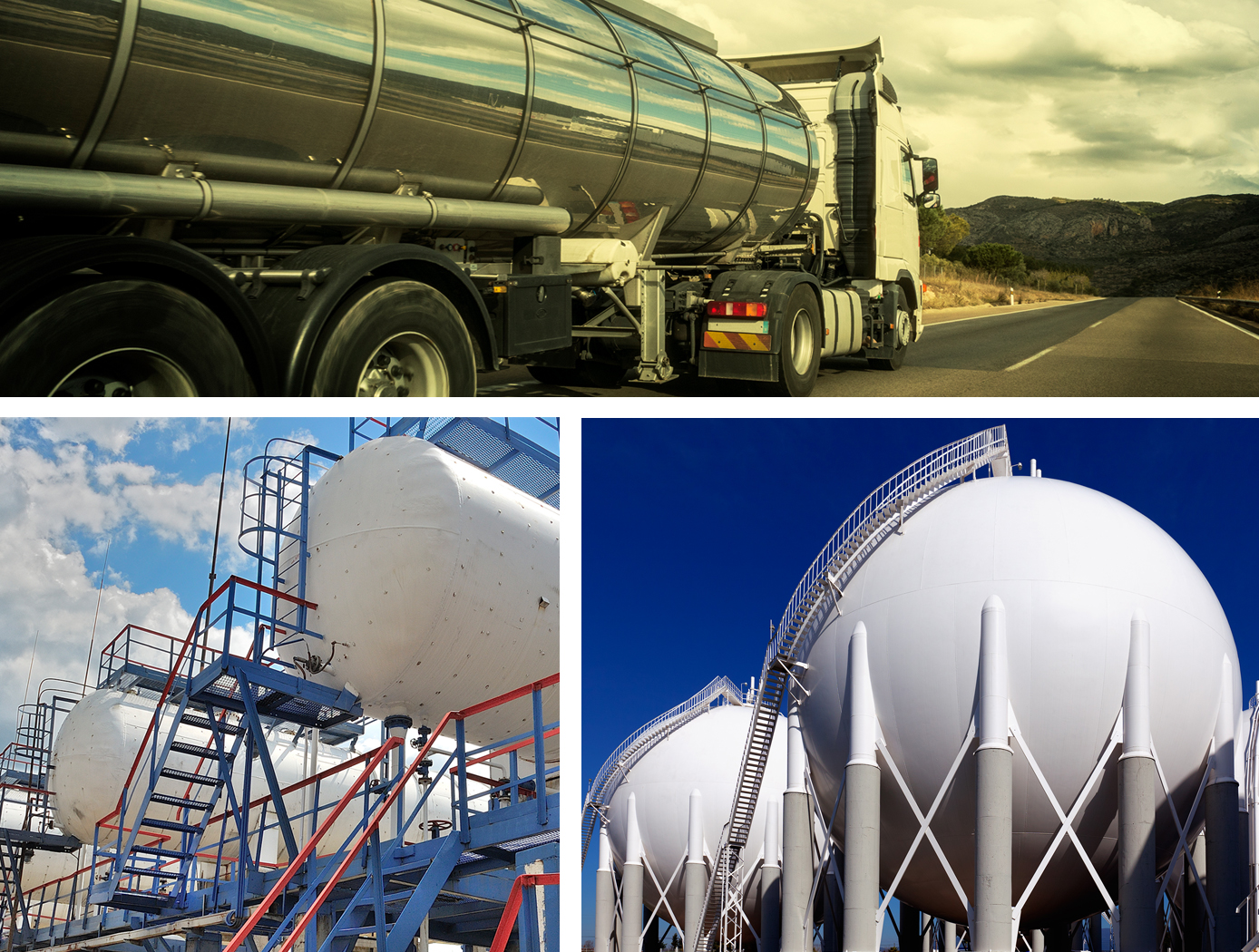
Benefits
- Robust: Provides a moisture-free and mechanically isolated condition for the sensing fibers
- Accurate: Offers excellent thermal conductivity to surrounding area, allowing the sensing fibers to determine temperature correctly
- Reliable: Enables fiber optic sensing systems to operate properly in humid environments
Applications
- Energy: Liquid natural gas and petrochemical storage tanks
- Industrial: Storing cryogenic liquids (e.g., liquid nitrogen)
- Aerospace: Liquid fuel tanks for launch vehicles
Technology Details
sensors
DRC-TOPS-25
DRC-016-022
Similar Results

Streamlined Liquid Level Sensing Using Fiber Optics
Armstrong has developed a robust fiber optic–based sensing technology that offers extraordinary accuracy in liquid level measurements. The sensing system uses fiber optic Bragg sensors located along a single fiber optic cable. These sensors actively discern between the liquid and gas states along a continuous fiber and can accurately pinpoint the liquid level.
How It Works
The technology uses a resistive heater wire bundled with the optical fiber. The heater is pulsed to induce a local temperature change along the fiber, and the fiber Bragg grating data is used to monitor the subsequent cooling of the fiber. The length of fiber in the liquid cools more rapidly than the portion of the fiber in the gas above the liquid. The measurement system accurately establishes the location of this transition to within 1/4-inch.
Why It Is Better
Armstrong's liquid level sensing technology was originally developed to measure cryogenic liquid levels in rockets, and it represents a significant advancement in the state of the art in this application. Conventional methods for measuring cryogenic liquid levels rely on cryogenic diodes strategically placed along a rod or rack. The diodes are mounted in pre-selected, relatively widely spaced positions along the length of a rod; this configuration provides limited, imprecise data. Furthermore, each diode on the rod has two wires associated with it, which means a single system may require a large number of wires, making installation, connectivity, and instrumentation cumbersome.
Armstrong's novel technology provides liquid measurements with much greater precision, achieving measurements at 1/4-inch intervals. Furthermore, the streamlined system uses just two wires, which greatly simplifies installation and instrumentation. Due to its extraordinary accuracy and ease of use, Armstrong's measurement system offers important advantages for a wide range of applications beyond cryogenic liquids.
In Addition
Researchers have developed a new manufacturing process that improves the ability of fiber optic sensing systems to measure temperature and liquid levels when operating in humid environments. The process involves eliminating moisture from the optical fiber coating, then completing the sensor assembly within humidity-controlled conditions. The resulting sensor hardware provides precise and accurate measurements even when operating in a humid environment.
For more information about the full portfolio of FOSS technologies, see DRC-TOPS-37 or visit https://technology-afrc.ndc.nasa.gov/featurestory/fiber-optic-sensing

Lightweight Fiber Optic Sensors for Real-Time Monitoring of Structural Health
How It Works
The FOSS technology employs efficient, real-time, data driven algorithms for interpreting strain data. The fiber Bragg grating sensors respond to strain due to stress or pressure on the substrate. The sensors feed these strain measurements into the systems algorithms to determine shape, stress, temperature, pressure, strength, and operational load in real time.
Why It Is Better
Conventional strain gauges are heavy, bulky, spaced at distant intervals (which leads to lower resolution imaging), and unable to provide real-time measurements. Armstrong's system is virtually weightless, and thousands of sensors can be placed at quarter-inch intervals along an optical fiber the size of a human hair. Because these sensors can be placed at such close intervals and in previously inaccessible regions (for example, within bolted joints, embedded in a composite structure), the high-resolution strain measurements are more precise than ever before. The fiber optic sensors are non-intrusive and easy to install—thousands of sensors can be installed in less time than conventional strain sensors and the system is capable of processing information at the unprecedented rate of 100 samples per second. This critical, real-time monitoring capability enables an immediate and informed response in the event of an emergency and allows for precise, controlled monitoring to help avoid such scenarios.
For more information about the full portfolio of FOSS technologies, see DRC-TOPS-37 or visit https://technology-afrc.ndc.nasa.gov/featurestory/fiber-optic-sensing

Fiber Optic Sensing Technologies
The FOSS technology revolutionizes fiber optic sensing by using its innovative algorithms to calculate a range of useful parameters—any and all of which can be monitored simultaneously and in real time. FOSS also couples these cutting-edge algorithms with a high-speed, low-cost processing platform and interrogator to create a single, robust, stand-alone instrumentation system. The system distributes thousands of sensors in a vast network—much like the human body's nervous system—that provides valuable information.
How It Works
Fiber Bragg grating (FBG) sensors are embedded in an optical fiber at intervals as small as 0.25 inches, which is then attached to or integrated into the structure. An innovative, low-cost, temperature-tuned distributed feedback (DFB) laser with no moving parts interrogates the FBG sensors as they respond to changes in optical wavelength resulting from stress or pressure on the structure, sending the data to a processing system. Unique algorithms correlate optical response to displacement data, calculating the shape and movement of the optical fiber (and, by extension, the structure) in real time, without affecting the structure's intrinsic properties. The system uses these data to calculate additional parameters, displaying parameters such as 2D and 3D shape/position, temperature, liquid level, stiffness, strength, pressure, stress, and operational loads.
Why It Is Better
FOSS monitors strain, stresses, structural instabilities, temperature distributions, and a plethora of other engineering measurements in real time with a single instrumentation system weighing less than 10 pounds. FOSS can also discern between liquid and gas states in a tank or other container, providing accurate measurements at 0.25-inch intervals. Adaptive spatial resolution features enable faster signal processing and precision measurement only when and where it is needed, saving time and resources. As a result, FOSS lends itself well to long-term bandwidth-limited monitoring of structures that experience few variations but could be vulnerable as anomalies occur (e.g., a bridge stressed by strong wind gusts or an earthquake).
As a single example of the value FOSS can provide, consider oil and gas drilling applications. The FOSS technology could be incorporated into specialized drill heads to sense drill direction as well as temperature and pressure. Because FOSS accurately determines the drill shape, users can position the drill head exactly as needed. Temperature and pressure indicate the health of the drill. This type of strain and temperature monitoring could also be applied to sophisticated industrial bore scope usage in drilling and exploration.
For more information about the full portfolio of FOSS technologies, see visit https://technology-afrc.ndc.nasa.gov/featurestory/fiber-optic-sensing

Adaptive Spatial Resolution Enables Focused Fiber Optic Sensing
This technology can be applied to most optical frequency domain reflectometry (OFDR) fiber optic strain sensing systems. It is particularly well suited to Armstrong's FOSS technology, which uses efficient algorithms to determine from strain data in real time a variety of critical parameters, including twist and other structural shape deformations, temperature, pressure, liquid level, and operational loads.
How It Works
This technology enables smart-sensing techniques that adjust parameters as needed in real time so that only the necessary amount of data is acquired—no more, no less.
Traditional signal processing in fiber optic strain sensing systems is based on fast Fourier transform (FFT), which has two key limitations. First, FFT requires having analysis sections that are equal in length along the whole fiber. Second, if high resolution is required along one portion of the fiber, FFT processes the whole fiber at that resolution. Armstrong's adaptive spatial resolution innovation makes it possible to efficiently break up the length of the fiber into analysis sections that vary in length. It also allows the user to measure data from only a portion of the fiber. If high resolution is required along one section of fiber, only that portion is processed at high resolution, and the rest of the fiber can be processed at the lower resolution.
Why It Is Better
To quantify this innovation's advantages, this new adaptive method requires only a small fraction of the calculations needed to provide additional resolution compared to FFT (i.e., thousands versus millions of additional calculations). This innovation provides faster signal processing and precision measurement only where it is needed, saving time and resources. The technology also lends itself well to long-term bandwidth-limited monitoring systems that experience few variations but could be vulnerable as anomalies occur.
More importantly, Armstrong's adaptive algorithm enhances safety, because it automatically adjusts the resolution of sensing based on real-time data. For example, when strain on a wing increases during flight, the software automatically increases the resolution on the strained part of the fiber. Similarly, as bridges and wind turbine blades undergo stress during big storms, this algorithm could automatically adjust the spatial resolution to collect more data and quickly identify potentially catastrophic failures.
This innovation greatly improves the flexibility of fiber optic strain sensing systems, which provide valuable time and cost savings to a range of applications.
For more information about the full portfolio of FOSS technologies, see DRC-TOPS-37 or visit https://technology-afrc.ndc.nasa.gov/featurestory/fiber-optic-sensing

Novel Solid-State Humidity Sensor
NASAs novel ceramic dielectric material enables extremely high-sensitivity humidity sensing. The ceramic sensing element is robust, can be manufactured using printing processes, and exhibits fast response and recovery speeds with large capacitance and resistance response/change per relative humidity unit change across a wide range of humidity levels in a log-linear response. Preliminary test data conducted in a humidity test chamber show a log-linear measured response in capacitance from 5 nanofarads (at 30% relative humidity, room temperature) to 0.2 millifarads (at 90% relative humidity, room temperature).
The inventors discovered the humidity sensing element technology during their efforts to develop next-generation energy storage materials and devices for NASA. The inventors were initially puzzled by large swings in capacitance observed over the course of any given day in one particular dielectric composition, and, ultimately, they were able to trace these unexpected changes in capacitance back to corresponding changes in ambient humidity, even those occurring from breathing and exhalation.
The sensor element can be formed using a dielectric ink or paste formulation, also developed by NASA, via traditional screen printing or advanced ink jet, aerosol, or 3D printing methods. The printed sensor element can be very thin, on the order of microns in thickness, with a small footprint, one square centimeter or less.