Low Creep, Low Relaxation Fiber-Reinforced Polymer Composites
materials and coatings
Low Creep, Low Relaxation Fiber-Reinforced Polymer Composites (LAR-TOPS-356)
Increased dimensional stability
Overview
Inflatable and deployable beams and masts such as solar sail supports used in space missions are often made of polymer composites and may be stored for one to two years in space before deployment. While stored, these polymer composites degrade on a molecular level, which can limit the ability of the beams to unfurl properly, reducing performance or even failing to unfurl. Researchers at NASAs Langley Research Center developed a fiber-reinforced polymer composite to reduce the effect of viscoelastic creep and prolong the molecular integrity of polymer-based beams over time. NASAs polymer composite technology augments the molecular structure of the resin matrix by incorporating secondary additives and adjusting the composite architecture. Test results show that the new material reduces relaxation in the modified-molecular structure to as low as 5% from the initial modulus after two years. This compares to 49% relaxation after one year for the state-of-the-art, commercially available options.
The Technology
NASA used three strategies to develop a family of modified epoxy resins for use in an improved composite layup configuration. By tailoring molecular structures, incorporating secondary additives, and adjusting composite architecture, NASA has produced fiber-reinforced polymer composites that suppress viscoelastic creep and relaxation. Specifically the three strategies were:
(1) Controlling the cross-linking density using reactive functional groups and selecting appropriate monomers with stoichiometry adjustments. A higher stoichiometric ratio is responsible for reduced relaxation compared to a lower ratio (resulting in 26% relaxation after 1 year vs. 49%).
(2) Increase the steric hindrance by reducing free volume and enhancing intermolecular interaction. This involved the preparation of two different reactive low molecular weight oligomers The best epoxy resin created was from the VCD/HAA additives with 15-17% relaxation; DEGBA/HAA had 22-25% relaxation.
(3) Optimizing different carbon fiber layup configurations for woven structures made into a highly stiff boom, highly flexible boom, a small size boom, and a large size boom application. The best configuration was that of a highly stiff boom with 4-5% relaxation. Other carbon fiber layup configurations had 16-30% relaxation.
As shown in the figure below, NASA tested the relaxed composite using an accelerated test with variable temperatures.
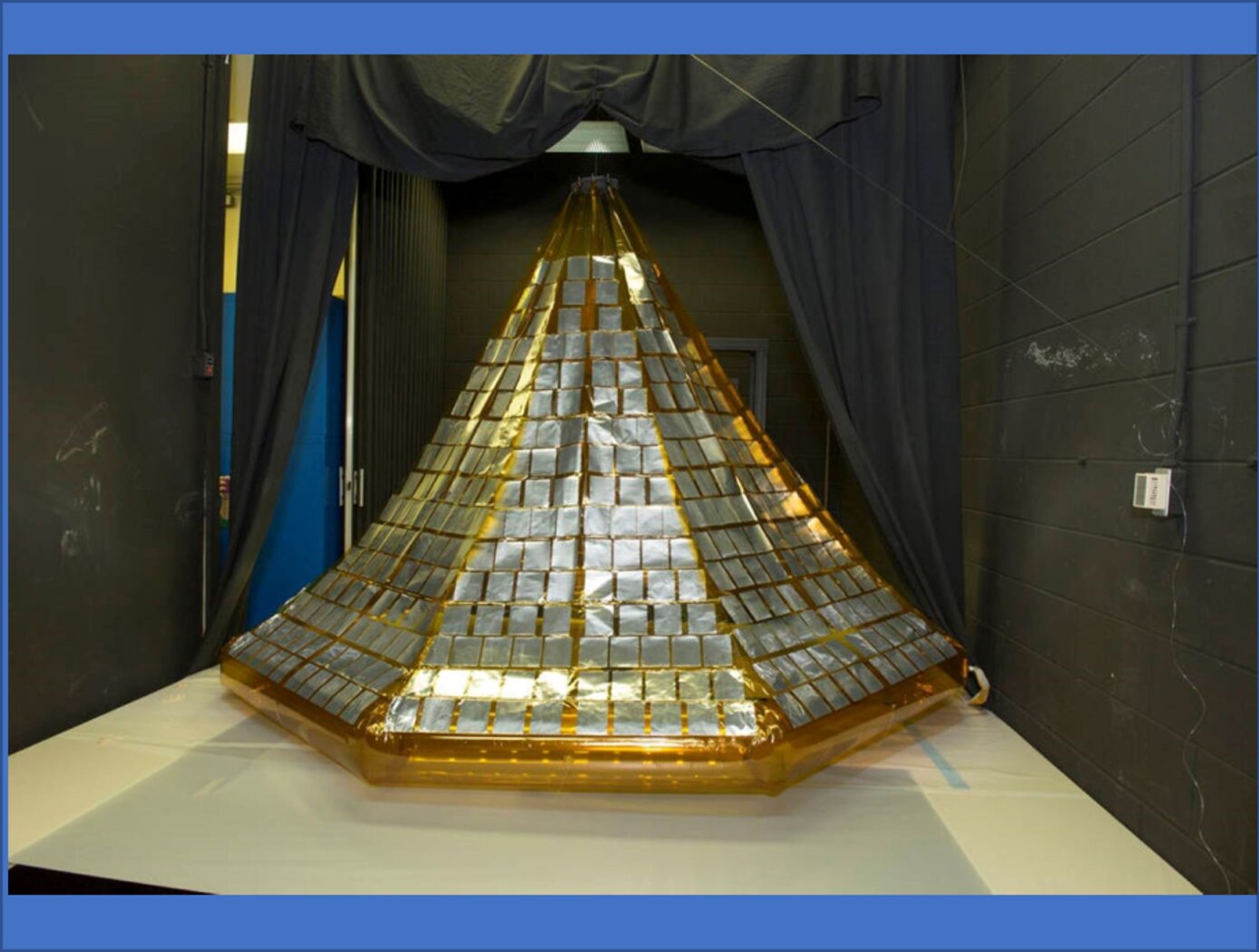

Benefits
- Improved Dimensional Stability: suppresses the onset of viscoelastic creep and relaxation
- Enhanced Properties: heightened specific strength and specific stiffness
- Variety: new polymeric materials and fiber-reinforced polymer composites
- Easy to Make: uses commercially available materials
Applications
- Aerospace: durable and stable inflatable and deployable structures (e.g., payload booms, solar sail deployable booms, solar power arrays, antenna supports, space habitats, planetary decelerators)
- Military: reinforced body armor for soldiers (e.g., vest, combat helmet, gloves), inflatable and deployable structures (e.g., barracks), reinforced shock-absorbing material for vehicles and aircraft, material for advanced combat weapons and tools
- Wind Energy: turbines
- Marine: hull boats in structural frames, keels, masts, poles, boom, winch drums, and shafting
- Sporting goods: improved durability and mechanical stability of a wide variety of sporting goods such as snow boards, golf clubs, tennis racquets, hockey sticks, etc.
Technology Details
materials and coatings
LAR-TOPS-356
LAR-19732-1
Similar Results

Origami-based Deployable Fiber Reinforced Composites
Deployable space structures often rely upon telescoping or folding structures that either must be manually deployed or deployed by attached motors. These structures are often made from heavier (relative to carbon fiber composites) metals to provide enough strength to support a load. As such, there is a need for in-space structures that are lightweight, can be packaged compactly, and can be deployed easily.
The composite material developed here does not require high temperature baking to cure the polymer, rather relying on UV light to solidify the polymer component. The composite is then included into origami-based structures that can fold and deploy using the polymer shape memory effect. The composite is first trained to assume the deployed structural shape when heated; it is then folded like origami and frozen into the packaged shape for storage and launch. Combining the composite material with the origami-inspired design leads to high strength structures (can hold at least 600 kg on Earth). To date, a ~5-inch prototype structural bar has been produced using the UV-curable composite and further development is on-going at NASA Langley.
The deployable origami composite structures are at technology readiness level (TRL) 4 (component and/or breadboard validation in laboratory environment) and are available for patent licensing.

Carbon Fiber-Carbon Nanotube Yarn Hybrid Reinforcement
NASA's new material is a toughened triaxial braid made from ductile carbon nanotube (CNT) yarn hybridized with carbon fiber, which is ultimately used as reinforcement material to make toughened polymer matrix composites. The CNT yarn component of the reinforcement is solely responsible for adding toughness, while the processes used to optimize the fiber braiding parameters and tensile properties of the carbon fiber-CNT yarn hybrid tow material determine the overall improvement in tensile strength for resin impregnated fiber tows. Bundles of continuous carbon nanotube yarns are combined with a similar format of carbon fiber, yielding an easily scalable process.
Advantages of the material include reduced cost by eliminating use of toughening agents, increased ability to conform to highly complex geometries, greater environmental stability compared to aramid fiber reinforcements such as Kevlar, and possibly decreased density. Many hybrid reinforcements exhibit interfacial compatibility issues, which could lead to premature failure via crack propagation at the polymer matrix interface. In contrast, chemical similarities between the CNT yarn and carbon fiber constituents impart NASA's hybrid reinforcement material with excellent interfacial compatibility.
Potential applications include aerospace components, composite pressure vessels, wind turbine blades, automotive components, prosthetics, sporting equipment, construction reinforcement material, and other use-cases where strength-to-weight ratio is of utmost importance.

Highly Thermal Conductive Polymeric Composites
There has been much interest in developing polymeric nanocomposites with ultrahigh thermal conductivities, such as with exfoliated graphite or with carbon nanotubes. These materials exhibit thermal conductivity of 3,000 W/mK measured experimentally and up to 6,600 W/mK predicted from theoretical calculations. However, when added to polymers, the expected thermal conductivity enhancement is not realized due to poor interfacial thermal transfer.
This technology is a method of forming carbon-based fillers to be incorporated into highly thermal conductive nanocomposite materials. Formation methods include treatment of an expanded graphite with an alcohol/water mixture followed by further exfoliation of the graphite to form extremely thin carbon nanosheets that are on the order of between about 2 and about 10 nanometers in thickness. The carbon nanosheets can be functionalized and incorporated as fillers in polymer nanocomposites with extremely high thermal conductivities.

Self-Healing Low-Melt Polyimides
There are multiple space-related systems that can benefit from high performance, thin film, self-healing/sealing systems. Space vehicles and related ground support equipment can contain miles of wire, much of which is buried inside structures making it very difficult to access for inspection and repair. Space-based inflatable structures, solar panels, and astronauts performing extra-vehicular activities are subject to being struck by micrometeoroids and orbital debris. Self-healing or sealing layers on inflatables, solar panels and spacesuits would increase the safety and survivability of astronauts as well as the survivability and functionality of inflatables and solar panels. Self-healing insulation on wiring would greatly improve the reliability and safety of systems containing such wiring and reduce inspection and repair time over the lifetime of those systems. This technology combines the use of a self-sealing low melt, high performance polyimide film that exhibits the ability, when cut, for separated edges to slowly flow back together and seal itself, with the options of a laminate system and the inclusion of healant microcapsules that, when broken, release healant which can then additionally assist in the healing process. Combinations of the healing approaches can be enabling to the healing process proceeding at a much greater rate and dual mode healing approach can also allow for healing of a larger area.

Durable Polyimide Aerogels
Aerogels are highly porous, low-density solids with extremely small pore sizes, fabricated by forming a gel from a solution in the wet-gel state that is then converted to the dry-solid state without compaction of the porous architecture. Aerogels make excellent electrical, thermal, and acoustic insulators. However, most inorganic silica aerogels are fragile and shed dust. The NASA Glenn team is the first to synthesize three-dimensional polymer aerogel networks of polyimides cross-linked with multifunctional amine monomers. Compared to silica aerogels, these aerogels retain small pore sizes and low thermal conductivities, but are distinguished by their flexibility. Polyimide aerogels are not brittle, fragile, or dusty like silica aerogels. Plus, polyimide aerogels possess the beneficial characteristics and strength of polyimide materials. The results are cross-linked polyimide aerogels with little shrinkage, low densities, high compression and tensile strengths, and good moisture resistance. They can be fabricated or machined into net shape parts, which are strong and stiff, or cast as thin flexible films with good tensile properties. Extremely customizable, polyimide aerogels can be formed into any configuration (e.g., wrapped around a pipe, sewn into protective clothing, or molded into a panel to act as a heat shield in a car). In short, Glenn's innovation improves the performance, adaptability, and affordability of aerogels in a broad number of applications.