eVTOL UAS with Lunar Lander Trajectory
Aerospace
eVTOL UAS with Lunar Lander Trajectory (LAR-TOPS-371)
Rapid, repeatable, & cost-effective EDL sensor testing on Earth
Overview
Lunar landers are critical to enabling NASA’s mission of sustained human presence on the Moon. These spacecraft require advanced landing technologies (e.g., sensors, algorithms, navigation loops) to enable automated and precise entry, descent, and landing (EDL) on planetary surfaces. EDL operations rely on advanced, high-accuracy sensors that enhance navigation precision and improve the control performance of the spacecraft. These sensors must undergo rigorous testing and validation on Earth before mission use.
To test EDL sensors today, organizations generally use helicopter or rocket flights to simulate lander trajectories. However, helicopters are not capable of achieving the rapid descents experienced by landers (due to vortex ring state). Rocket flights are expensive, high risk, often not repeatable, and cannot be performed in rapid succession. This limits the volume and quality of data available for EDL sensor verification and validation, making iterative testing and development slower and lower fidelity than desired.
In response to this challenge, engineers at NASA’s Langley Research Center (LaRC) have developed an electric vertical takeoff and landing (eVTOL) unmanned aerial system (UAS) designed to fly trajectories with high similitude to those flown by lunar landers. The NASA invention is poised to enable rapid development and testing of EDL sensors and improve EDL sensor testing data quality at a fraction of the cost of alternative methods.
The Technology
This NASA-developed eVTOL UAS is a purpose-built, electric, reusable aircraft with rotor/propeller thrust only, designed to fly trajectories with high similarity to those flown by lunar landers. The vehicle has the unique capability to transition into wing borne flight to simulate the cross-range, horizontal approaches of lunar landers. During transition to wing borne flight, the initial transition favors a traditional airplane configuration with the propellers in the front and smaller surfaces in the rear, allowing the vehicle to reach high speeds. However, after achieving wing borne flight, the vehicle can transition to wing borne flight in the opposite (canard) direction. During this mode of operation, the vehicle is controllable, and the propellers can be powered or unpowered.
This NASA invention also has the capability to decelerate rapidly during the descent phase (also to simulate lunar lander trajectories). Such rapid deceleration will be required to reduce vehicle velocity in order to turn propellers back on without stalling the blades or catching the propeller vortex. The UAS also has the option of using variable pitch blades which can contribute to the overall controllability of the aircraft and reduce the likelihood of stalling the blades during the deceleration phase.
In addition to testing EDL sensors and precision landing payloads, NASA’s innovative eVTOL UAS could be used in applications where fast, precise, and stealthy delivery of payloads to specific ground locations is required, including military applications. This concept of operations could entail deploying the UAS from a larger aircraft.
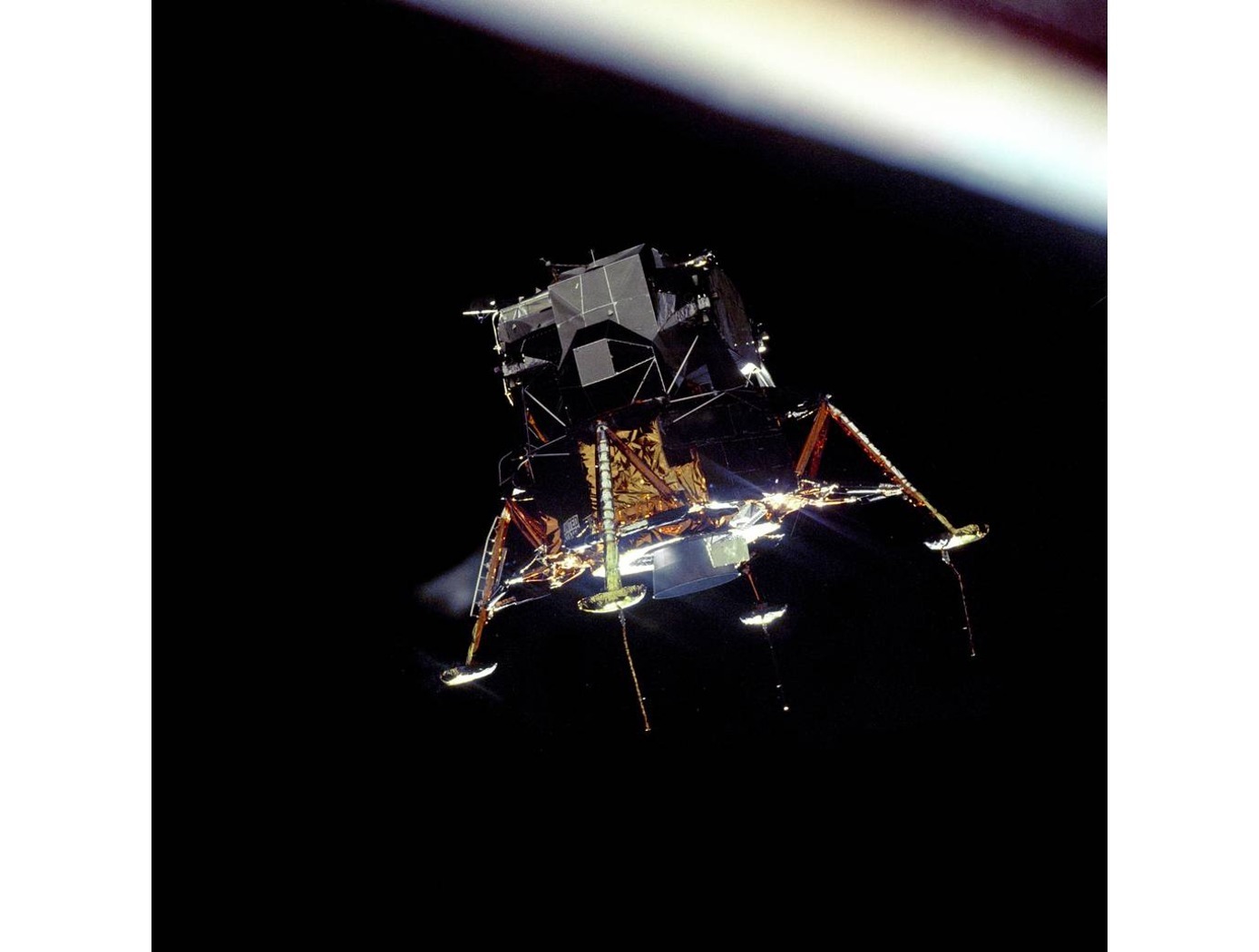
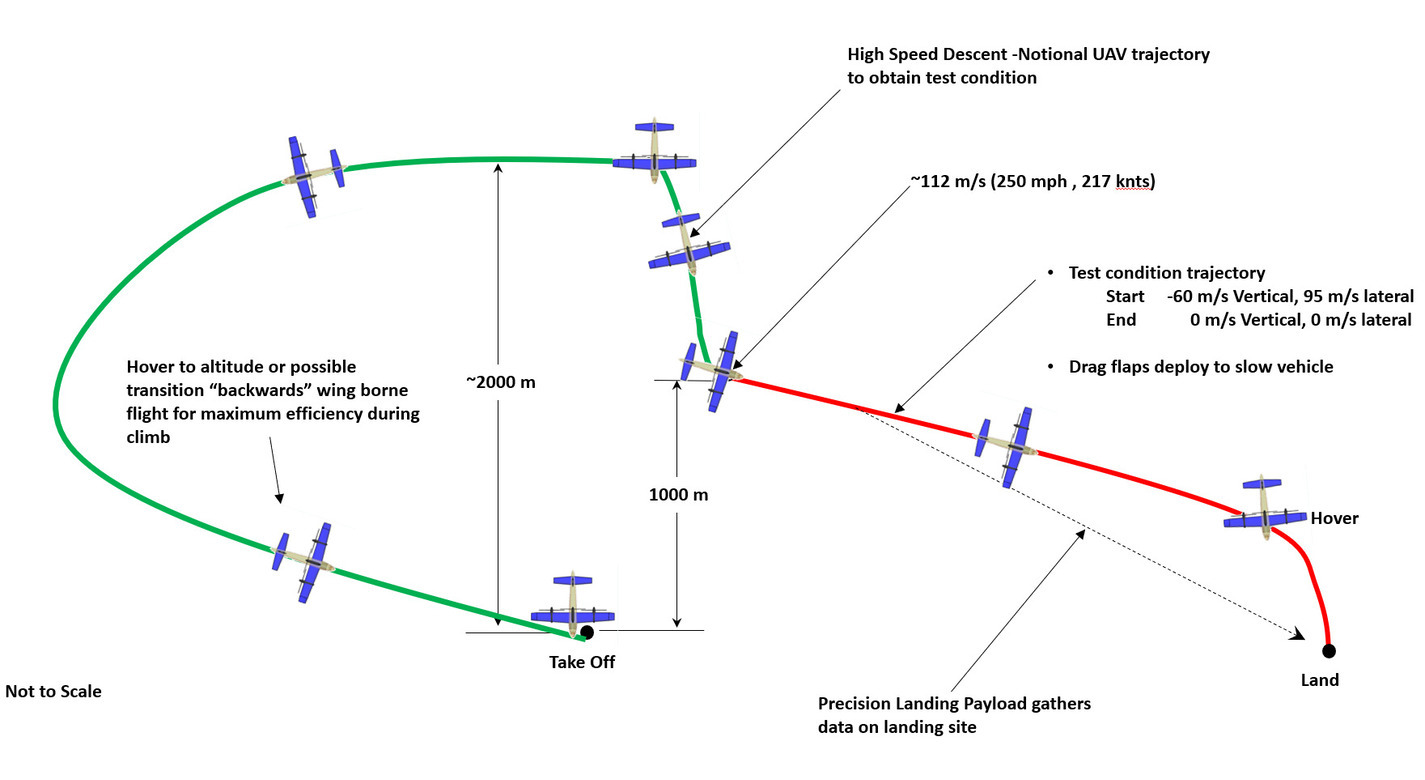
Benefits
- Provides improved EDL sensor testing data: NASA’s UAS was designed to closely match lunar lander trajectories and operational conditions on Earth. More representative flights tests improve the quality of testing data.
- Reduces EDL sensor flight test costs: Provides significantly cheaper testing relevant to (lower fidelity) alternatives such as helicopter and rocket testing.
- Enables increased test flight volume: This innovative UAS is designed to be all-electric and reusable, meaning it could be flown 10+ times per day. Thus, the development-test-validation feedback loop for EDL sensors can be significantly shortened, allowing for rapid iterative development.
- Capable of rapid deceleration: Due to its unique design and control system, the UAS is capable of achieving stable and controlled flight states as it passes through the vortex region of flow, unlike alternative flight vehicles such as helicopters.
- Adaptable for other propulsion technologies: While designed to be all-electric, the UAS could be manufactured for hybrid-electric or internal combustion power sources for applications requiring increased range.
Applications
- Lunar lander EDL sensor testing & validation: NASA’s UAS was developed to enable trajectories with high similitude to those experienced by lunar landers (improving EDL test data quality), reduce EDL testing flight costs, and increase feasible flight tests per day given its reusable design.
- Fast, precise, and stealthy delivery of payloads to ground locations: Given its unique trajectory capabilities (e.g., ability to achieve rapid deceleration while mitigating vortex ring states), the system could be used to deliver payloads with high precision and speed to desired ground locations. For such use-cases, the VTOL UAS could be deployed from a larger aircraft, immediately entering the horizontal, cross-range portion of its trajectory.
Technology Details
Aerospace
LAR-TOPS-371
LAR-20212-1
Tags:
|
Similar Results

Unique Datapath Architecture Yields Real-Time Computing
The DLC platform is composed of three key components: a NASA-designed field programmable gate array (FPGA) board, a NASA-designed multiprocessor on-a-chip (MPSoC) board, and a proprietary datapath that links the boards to available inputs and outputs to enable high-bandwidth data collection and processing.
The inertial measurement unit (IMU), camera, Navigation Doppler Lidar (NDL), and Hazard Detection Lidar (HDL) navigation sensors (depicted in the diagram below) are connected to the DLC’s FPGA board. The datapath on this board consists of high-speed serial interfaces for each sensor, which accept the sensor data as input and converts the output to an AXI stream format. The sensor streams are multiplexed into an AXI stream which is then formatted for input to a XAUI high speed serial interface. This interface sends the data to the MPSoC Board, where it is converted back from the XAUI format to a combined AXI stream, and demultiplexed back into individual sensor AXI streams. These AXI streams are then inputted into respective DMA interfaces that provide an interface to the DDRAM on the MPSoC board. This architecture enables real-time high-bandwidth data collection and processing by preserving the MPSoC’s full ability.
This sensor datapath architecture may have other potential applications in aerospace and defense, transportation (e.g., autonomous driving), medical, research, and automation/control markets where it could serve as a key component in a high-performance computing platform and/or critical embedded system for integrating, processing, and analyzing large volumes of data in real-time.

Low Weight Flight Controller Design
Increasing demand for smaller UAVs (e.g., sometimes with wingspans on the order of six inches and weighing less than one pound) generated a need for much smaller flight and sensing equipment. NASA Langley's new sensing and flight control system for small UAVs includes both an active flight control board and an avionics sensor board. Together, these compare the status of the UAVs position, heading, and orientation with the pre-programmed data to determine and apply the flight control inputs needed to maintain the desired course.
To satisfy the small form-factor system requirements, micro-electro-mechanical systems (MEMS) are used to realize the various flight control sensing devices. MEMS-based devices are commercially available single-chip devices that lend themselves to easy integration onto a circuit board. The system uses less energy than current systems, allowing solar panels planted on the vehicle to generate the systems power. While the lightweight technology was designed for smaller UAVs, the sensors could be distributed throughout larger UAVs, depending on the application.

Improved Ground Collision Avoidance System
This critical safety tool can be used for a wider variety of aircraft, including general aviation, helicopters, and unmanned aerial vehicles (UAVs) while also improving performance in the fighter aircraft currently using this type of system.
Demonstrations/Testing
This improved approach to ground collision avoidance has been demonstrated on both small UAVs and a Cirrus SR22 while running the technology on a mobile device. These tests were performed to the prove feasibility of the app-based implementation of this technology. The testing also characterized the flight dynamics of the avoidance maneuvers for each platform, evaluated collision avoidance protection, and analyzed nuisance potential (i.e., the tendency to issue false warnings when the pilot does not consider ground impact to be imminent).
Armstrong's Work Toward an Automated Collision Avoidance System
Controlled flight into terrain (CFIT) remains a leading cause of fatalities in aviation, resulting in roughly 100 deaths each year in the United States alone. Although warning systems have virtually eliminated CFIT for large commercial air carriers, the problem still remains for fighter aircraft, helicopters, and GAA.
Innovations developed at NASAs Armstrong Flight Research Center are laying the foundation for a collision avoidance system that would automatically take control of an aircraft that is in danger of crashing into the ground and fly it—and the people inside—to safety. The technology relies on a navigation system to position the aircraft over a digital terrain elevation data base, algorithms to determine the potential and imminence of a collision, and an autopilot to avoid the potential collision. The system is designed not only to provide nuisance-free warnings to the pilot but also to take over when a pilot is disoriented or unable to control the aircraft.
The payoff from implementing the system, designed to operate with minimal modifications on a variety of aircraft, including military jets, UAVs, and GAA, could be billions of dollars and hundreds of lives and aircraft saved. Furthermore, the technology has the potential to be applied beyond aviation and could be adapted for use in any vehicle that has to avoid a collision threat, including aerospace satellites, automobiles, scientific research vehicles, and marine charting systems.

Real-Time, High-Resolution Terrain Information in Computing-Constrained Environments
NASA Armstrong collaborated with the U.S. Air Force to develop algorithms that interpret highly encoded large area terrain maps with geographically user-defined error tolerances. A key feature of the software is its ability to locally decode and render DTMs in real time for a high-performance airplane that may need automatic course correction due to unexpected and dynamic events. Armstrong researchers are integrating the algorithms into a Global Elevation Data Adaptive Compression System (GEDACS) software package, which will enable customized maps from a variety of data sources.
How It Works
The DTM software achieves its high performance encoding and decoding processes using a unique combination of regular and semi-regular geometric tiling for optimal rendering of a requested map. This tiling allows the software to retain important slope information and continuously and accurately represent the terrain. Maps and decoding logic are integrated into an aircraft's existing onboard computing environment and can operate on a mobile device, an EFB, or flight control and avionics computer systems. Users can adjust the DTM encoding routines and error tolerances to suit evolving platform and mission requirements. Maps can be tailored to flight profiles of a wide range of aircraft, including fighter jets, UAVs, and general aviation aircraft.
The DTM and GEDACS software enable the encoding of global digital terrain data into a file size small enough to fit onto a tablet or other handheld/mobile device for next-generation ground collision avoidance. With improved digital terrain data, aircraft could attain better performance. The system monitors the ground approach and an aircraft's ability to maneuver by predicting several multidirectional escape trajectories, a feature that will be particularly advantageous to general aviation aircraft.
Why It Is Better
Conventional DTM encoding techniques used aboard high-performance aircraft typically achieve relatively low encoding process ratios. Also, the computational complexity of the decoding process can be high, making them unsuitable for the real-time constrained computing environments of high-performance aircraft. Implementation costs are also often prohibitive for general aviation aircraft. This software achieves its high encoding process ratio by intelligently interpreting its maps rather than requiring absolute retention of all data. For example, the DTM software notes the perimeter and depth of a mining pit but ignores contours that are irrelevant based on the climb and turn performance of a particular aircraft and therefore does not waste valuable computational resources. Through this type of intelligent processing, the software eliminates the need to maintain absolute retention of all data and achieves a much higher encoding process ratio than conventional terrain-mapping software. The resulting exceptional encoding process allows users to store a larger library of DTMs in one place, enabling comprehensive map coverage at all times. Additionally, the ability to selectively tailor resolution enables high-fidelity sections of terrain data to be incorporated seamlessly into a map.

Lunar Surface Manipulation System
NASA Langley developed the LSMS because of the need for a versatile system capable of performing multiple functions on the lunar surface, such as unloading components from a lander, transporting components to an operational site and installing them, and supporting service and replacement during component life. Current devices used for in-space operations are designed to work on orbit (zero g) only and thus do not have sufficient strength to operate on planetary surfaces. Traditional cranes are specialized to the task of lifting and are not capable of manipulator-type positioning operations.
The innovations incorporated into the LSMS allow it to lower payloads to the ground over a significant portion of the workspace without use of a hoist, functioning like a robot manipulator, thus providing a rigid connection and very precise control of the payload. The LSMS uses a truss architecture with pure compression and tension members to achieve a lightweight design. The innovation of using multiple spreaders (like spokes in a wheel) allows the LSMS to maintain its high structural efficiency throughout its full range of motion. Rod portions of the tension members automatically lift off and re-engage the spreaders as the joint articulates, allowing a large range of motion while maintaining mechanical advantage. In addition, the LSMS uses a quick-change device at the tip end that enables automated acquisition of end effectors or special purpose tools to increase its versatility.