Ruthenium-Doped Thermoelectric Materials
materials and coatings
Ruthenium-Doped Thermoelectric Materials (LEW-TOPS-139)
Robust material converts excess heat energy into electricity
Overview
Innovators at NASA's Glenn Research Center have developed a novel thermoelectric material that raises the bar for solid-state power conversion devices. There is growing momentum in the aerospace and automotive industries to harvest energy from heat (such as exhaust from combustion), but advances have been hampered by the lack of environmental durability and performance levels of thermoelectric materials currently in use. Glenn's breakthrough material is a ruthenium-doped gadolinium orthotantalate that excels at directly converting heat into energy. More important, this material does not break down at higher temperatures or air environments - even without special coatings or inert packaging. Glenn's pioneering material enables designers to make great strides in developing solid-state power conversion devices for application in aerospace, automotive, and power-generation industries.
The Technology
Solid state power conversion devices, such as thermoelectrics, depend upon temperature gradients for their operation. For example, aeronautic gas turbine engines maintain the necessary temperature gradients throughout their systems due to the enthalpic processes of combustion, which offers the possibility of generating electrical power for use in primary and secondary electrical systems in the aircraft. However, until now thermoelectric materials have not been able to withstand the combination of high temperatures and oxidative environments present in gas turbine engines. Glenn's innovation overcomes these limitations by using a doped oxide pyrochlore (crystal compound) semiconductor as the thermoelectric material. The material has a low thermal conductivity, which allows it to maintain a thermal gradient and sufficient electrical conductivity to produce an electromotive force. The pyrochlore allows the thermoelectric material to be present within a gas turbine engine, converting heat directly into electricity and functioning at high temperatures without oxidizing in air. Glenn's innovative thermoelectric material permits the benefits of solid-state power conversion devices to improve fuel efficiencies for a broader range of applications than has ever been possible. This innovation is in the early stages of development, and Glenn welcomes opportunities for co-development.
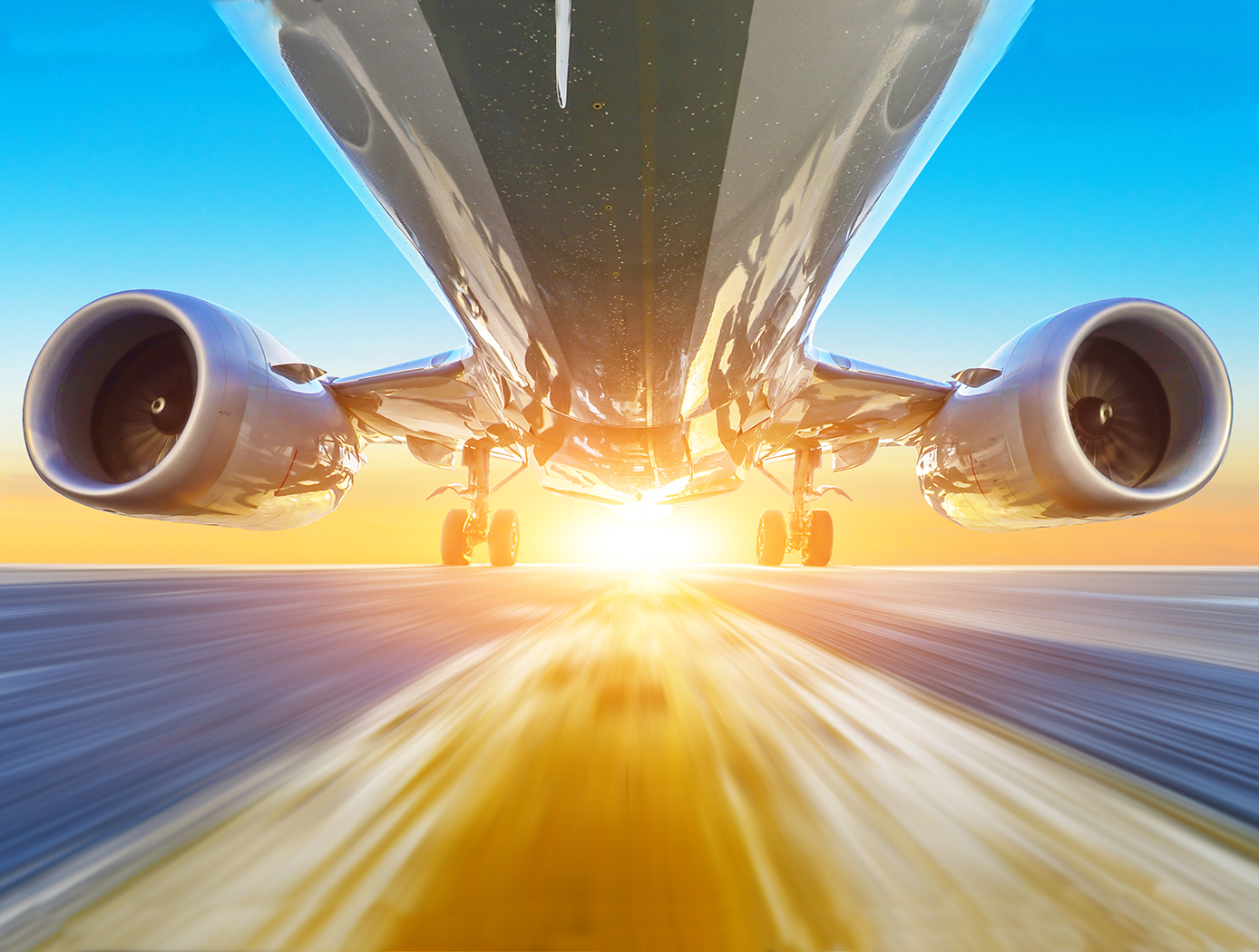
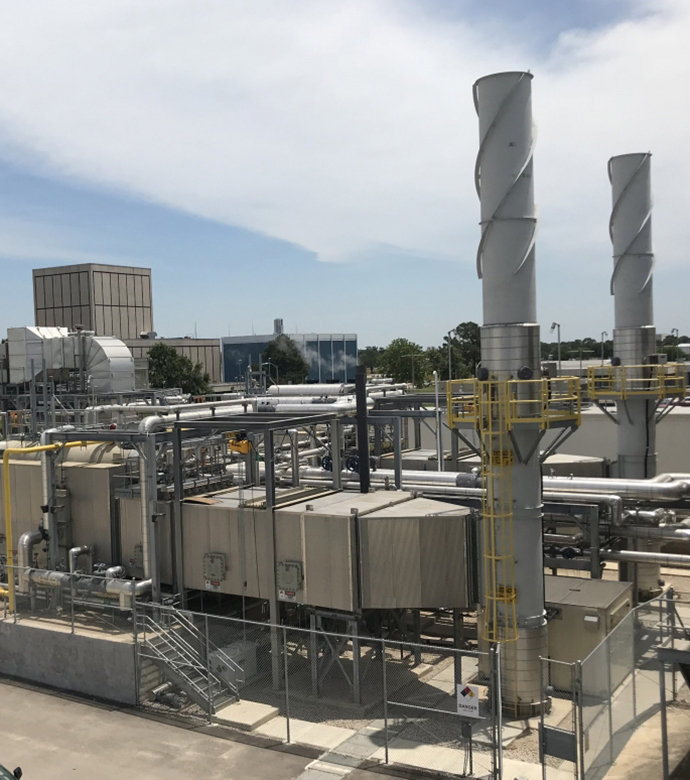
Benefits
- Efficient: Can convert waste enthalpy (such as exhaust) into electrical power in gas turbine engines
- Low Thermal Conductivity: Has a thermal conductivity of approximately 2W/mK, making this material an excellent thermoelectric
- Durable: Operates at temperatures above 700 degrees C, where current materials become unstable
- Oxidation-resistant: Does not oxidize in air, unlike current state-of-the-art thermoelectric materials
- Simple: Does not require special coatings or inert packaging
Applications
- Aerospace
- Automotive
- Electronics
- Power (e.g. Combined Heat and Power (CHP) systems)
- Semiconductors
- Turbines
- Unmanned vehicles
Similar Results

Stirling Thermoacoustic Power Converter and Magnetostrictive Alternator
Glenn's thermoacoustic power converter reshapes the conventional Stirling engine from a toroidal shape into a straight colinear arrangement. Instead of relying on failure-prone mechanical inertance and compliance tubes, this design achieves acoustical resonance by using electronic components. In a typical Stirling engine, the acoustical wave travels around a toroid and reflects back, forming a standing wave. In Glenn's device, by contrast, the wave instead travels in a straight plane where a transducer receives the acoustical wave and electrical components modulate the signal. A second transducer on the diametrically opposed side reintroduces the acoustic wave with the correct phasing to achieve amplification and resonance. Glenn's design allows the transducers to operate at high frequency while presenting a mass rather than stiffness impedance.
Glenn's magnetostrictive alternator uses stacked magnetostrictive materials under a biased magnetic and stress-induced compression. The acoustic energy from the engine travels through an impedance-matching layer (which can be formed from aerogel materials) that is physically connected to the magnetostrictive mass. Compression bolts keep the structure under compressive strain, allowing for the micron-scale compression of the magnetostrictive material and eliminating the need for bearings. The alternating compression and expansion of the magnetostrictive material creates an alternating magnetic field that then induces an electric current in a coil wound around the stack. This alternator produces electrical power from the acoustic pressure wave and, when the resonant frequency is tuned to match the engine, can replace the linear alternator to great effect.

Portable Compact Thermionic Power Cell
This compact thermionic cell (CTI) technology can be manufactured efficiently and economically using existing semiconductor fabrication technology. Its design consists of a top electron collector, separated by a vacuum gap from an electron emitter adjacent to the heat source, a thin plate of 238Pu enclosed by a thin-film insulator to protect the emitter and collector layers from overheating by the 238Pu. For a smart phone battery size, the invented compact thermionic (CTI) cell requires about 5 g maximum of 238Pu. Such small quantities are more readily available and producible, and could be reused for recycling when the CTI cell is dismantled. The emitter surface is topologically modified to have array of spikes, achievable using current semiconductor microfabrication technology. Various other geometries of emitter plates may also be used, such as an array of ridges. The smaller the emitter tips, the higher the voltage concentration.

Thermal Management for Aircraft Propulsion Systems
Aircraft thermal management systems typically comprise over half the mass associated with full electric power propulsion systems, with significant negative impact on fuel efficiency. In addition, the traditional method of using jet fuel to cool aircraft generators does not provide enough cooling for use in flight-weight cryogenic systems. Lastly, the much higher bus voltages required for flight-weight systems (4.5 kV vs. 270 V) introduce additional spark-ignition hazards associated with alternative cryogenic cooling fuels, including liquid methane or liquid hydrogen. The Glenn flight-weight thermal management system addresses all of these problems by using the considerable waste heat energy from turbogenerators to create a pressure wave thermoacoustically. This wave can then be delivered quietly and efficiently via routed ductwork to hollow pulse-tube coolers located near any component in the aircraft that requires cooling. The tubes can be fabricated in any length and can be curved to fit any space. This technology also allows waste heat energy to be used in at least four ways: 1) the waste heat energy can drive a thermoacoustics-based ambient or cryogenic heat pump; 2) it can be channeled directly into a thermoacoustic engine that generates power; 3) it can convectively preheat the fuel/ or air supplied to the aircraft engine; 4) it can drive a pulse-tube generator providing power. The delivered thermoacoustic power can provide cabin cooling as well as ambient/cryogenic cooling of converter, cables, and motors. In addition, this power can be converted to local electric power through the use of a transducer (such as a linear alternator) or piezoelectrics. Further, the efficient thermal management system enables the size, mass, and resultant cost of the radiating fins to be reduced. Glenn's system offers an efficient method of cooling next-generation flight-weight electric aircraft with significant benefits for fuel efficiency and safety.

Metallic Junction Thermoelectric (MJTE) Generator
The new TE device is fundamentally different from the conventional TE model in terms of high power density TE devices (at least > 30 W/kg) which is at least four times better than the radio-isotope thermoelectric generator (RTG). Innovations include:
•Does not use any junction technology of n-type and p-type which imposes the Brillouin limit due to the anisotropic electron plasma at the interface between n- and p-types.
•Does not manipulate and reduce the thermal conductivity of materials by tweaking morphological order of TE materials, which eventually causes a choking effect on thermal energy input into the system domain.
•Built with thin-film structure. An array structure in plane is fabricated by a simple micro-fabrication process and tied up with another layer built on top of its original layer embodiment as a tandem mode.
The TE device forms a tandem mode of multiple layers of thin-film TE array structure as repeated regenerative cycles to capture and convert more energy for high efficiency operation.

Nuclear Thermionic and Thermoelectric Energy System
The NASA patented NTAC-TE system is a promising technology for power generation from radioactive isotopes that generate gamma rays. This will reduce the mass of unusable radioactive waste products, as NTAC-TE can use what is normally waste and harmful in order to generate electric power.
The patented integration of Cobalt-59 into fission process, which will be transmuted into Co-60, with NTAC-TE elevates the efficiency well beyond current energy harvesting methodologies considering NTAC-TE can also harness energy from Cesium-137. The gamma radiation energies from these three sources combined together are 7 MeV from the prompt fission reaction, 0.6617 MeV from the Cesium by-product of the fission process, and 1.3325 MeV from Cobalt.