Portable Unit for Metabolic Analysis (PUMA)
health medicine and biotechnology
Portable Unit for Metabolic Analysis (PUMA) (LEW-TOPS-16)
Detects hypoxia to ensure health and safety of astronauts, pilots, divers, and miners
Overview
NASA's Glenn Research Center has developed the Portable Unit for Metabolic Analysis (PUMA) to provide highly precise real-time measurements of human metabolic functions. PUMA is a battery-powered, wearable device that measures concentrations of carbon dioxide and oxygen in inhaled and exhaled breath as well as heart rate, temperature, gas pressure, and inhalation and exhalation airflow rates. The device relays data wirelessly to a laptop computer for real-time analysis. Because the technology is packaged into a compact and wearable unit and can be used anywhere, a multitude of applications are possible, from ensuring the health and safety of astronauts, pilots, divers, and miners to monitoring patients with pulmonary disease and evaluating fitness levels of soldiers and athletes.
The Technology
PUMA represents a major breakthrough in portable metabolic analysis. It is a rugged, compact device that measures human metabolic function at rest, during exercise, in clinical settings, and in extreme environments. Metabolic measurements are a clinically proven method of monitoring cardiovascular health and fitness levels.
The PUMA headgear features NASA-developed sensors that evaluate six key metabolic functions. Specifically, PUMA measures oxygen and carbon dioxide partial pressure in addition to temperature, pressure, airflow, and heart rate. By placing sensors close to the mouth, PUMA can record up to 30 (or more) detailed measurements for each breath. From these measurements, PUMA computes metabolically relevant quantities of oxygen uptake, carbon dioxide output, minute ventilation, respiration rate, and heart rate. With additional software, the device computes heart rhythm, tidal volume, and alveolar and dead-space volumes. A small embedded computer controls and acquires data from all sensors at 10 hertz (Hz), performs calculations, and transmits data wirelessly to a remote computer. The PUMA sensors are low power, stable, and capable of operating in a range of environments, including very high and low pressures as well as high- and low-oxygen environments. This portable device provides real-time measurements that are just as accurate as the large stationary metabolic carts used in hospitals. PUMA can be used not only in clinical settings but also in the extreme/remote environments of space, aviation, underwater, and deep underground. Because it detects real-time dangerous drops in oxygen, it can ensure astronaut cardiovascular health; predict the onset of hypoxia in pilots, divers, and first responders; and advance chronic pulmonary disease monitoring and athletic training.
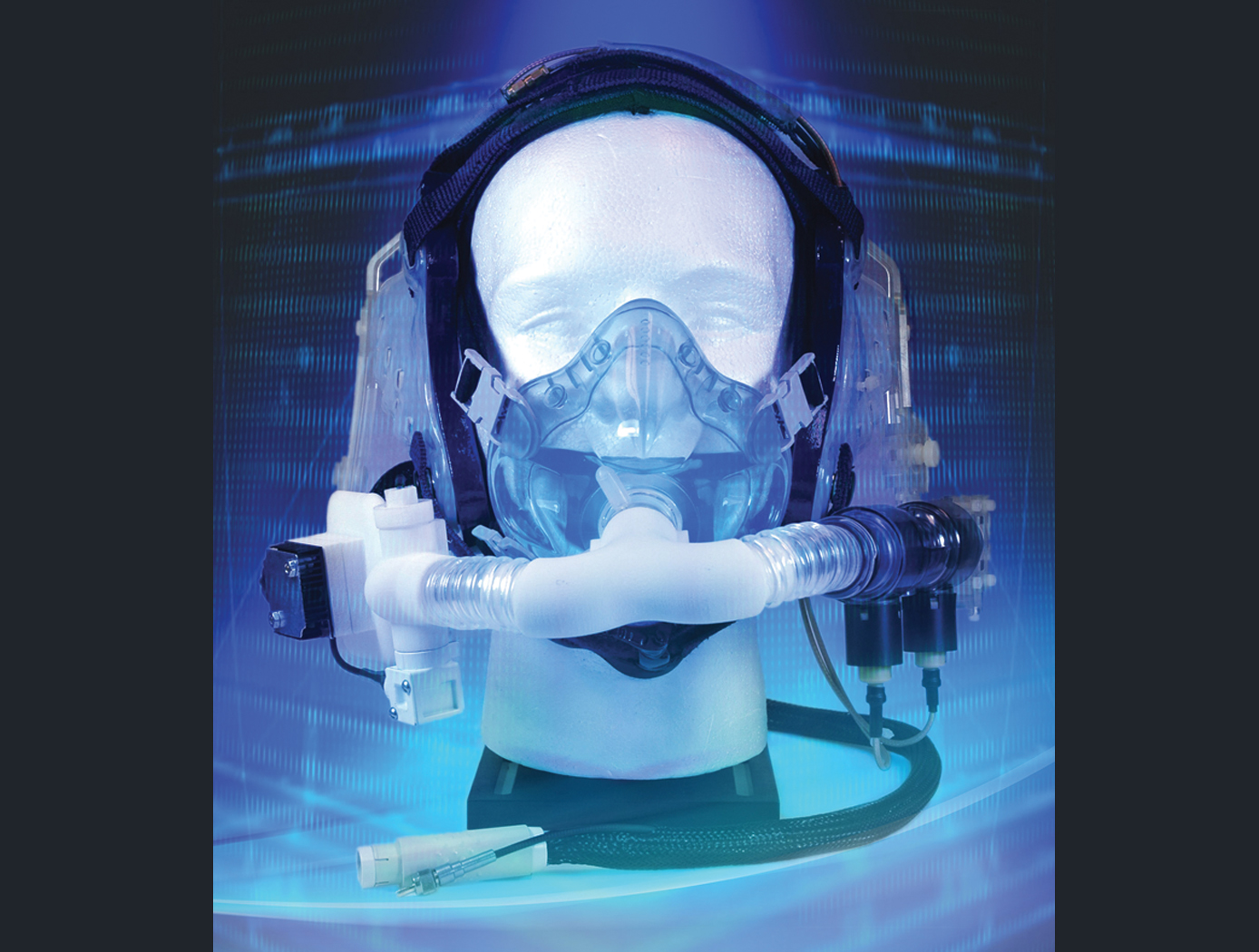
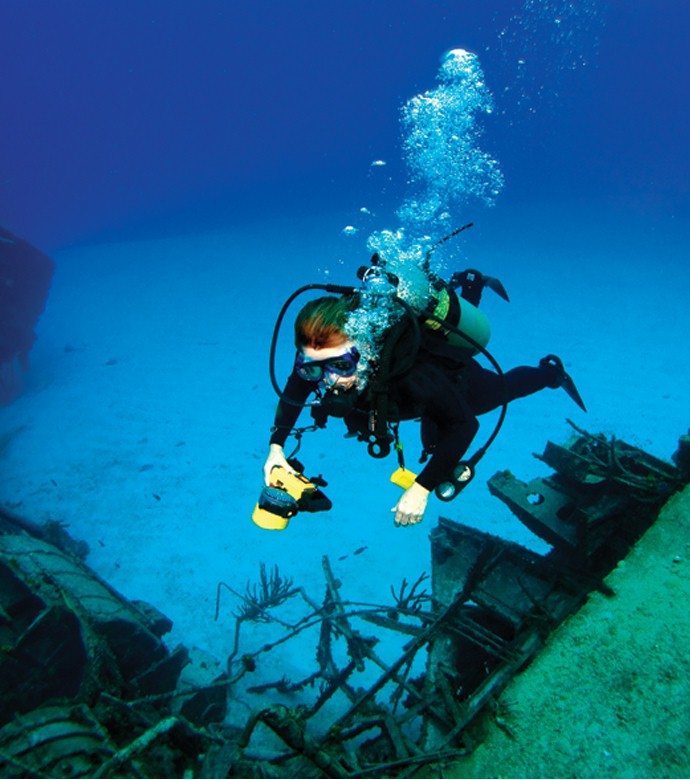
Benefits
- Highly Sensitive: PUMA is a unique portable metabolic unit that makes multiple real-time measurements per breath and samples oxygen and carbon dioxide close to the mouth.
- Predicts Hypoxia: The device is the first portable metabolic unit that senses and predicts the onset of hypoxia, a dangerous condition that results from inadequate oxygen.
- Proven: The technology has been validated in F-22 flight tests, and data was used to correlate flight information and aircraft performance with pilot physiological outputs.
- Versatile: Because of its unique design and innovative sensor technology, PUMA can be used to ensure astronaut health; detect hypoxia in pilots, divers, and first responders; and help physicians monitor chronic pulmonary disease.
- Fast: The response time of the PUMA technology is 10 milliseconds (ms), as compared to the 80120 ms of competing units. Faster response means more time to react to life-threatening situations.
Applications
- Health monitoring (hypoxia, extreme conditions)
- First responders
- Military
- Commercial space
- Wearables
- Health and wellness
Similar Results

Nanosensor Array for Medical Diagnoses
Many diseases are accompanied by characteristic odors. Their recognition can provide diagnostic clues, guide the laboratory evaluation, and affect the choice of immediate therapy. The study of the chemical composition of human breath using gas chromatography mass spectrometry (GC/MS) has shown a correlation between the volatile compounds and the occurrence of certain illnesses. The presence of those specific compounds can provide an indication of physiological malfunction and support the diagnosis of diseases. This condition requires an analytical tool with very high sensitivity for its measurement. A number of volatile compounds, so called biomarkers, are found in breath samples, normally at low parts per billion (ppb) levels. For example, the acetone in the exhaled breath from human with other biomarkers can indicate Type I diabetes. Usually, the concentration of the volatile compounds in human breath is very low and the background relative humidity is high, almost 100%. NASAs invention utilizes an array of chemical sensors combined with humidity, temperature, and pressure for real-time breath measurement to correlate the chemical information in the breath with the state and functioning of different human organs. This tool provides a non-invasive method for fast and accurate diagnosis at the medical point of care or at home. The sensor chip includes multisensors for a comprehensive measurement of chemical composition, temperature, humidity, and pressure/flow rate. The sensor data collected from this chip can be wired or wirelessly transmitted to a computer terminal at the doctors desk or hospital monitoring center. The sensor chip can be connected directly or via Universal serial bus (USB) to a cell phone for data transmission over a long distance and receive an instruction from a doctors office for an immediate therapy.

Polymer Electrolyte-Based Ambient Temperature Oxygen Microsensor
Conventional ambient-temperature oxygen sensors are limited in various ways: optically based sensors can be expensive and challenging to manufacture; electrochemical cells with liquid electrolytes can have limited lifetimes and become leak sources; and both types of sensors are difficult to miniaturize. These problems are addressed with Glenn's novel ambient temperature oxygen microsensor, which is based on a Nafiontm polymer electrolyte, microfabricated using thin-film technologies. In the past, one drawback of Nafiontm film has been that it can lose conductivity when the moisture content in the film is too low, potentially affecting sensor operation. Glenn researchers devised a method to use certain salts to hold water molecules in the Nafiontm film structure at room temperature. The presence of these salts provides extra sites in the film to promote proton (H+) mobility, thus improving film conductivity and overall sensor performance, particularly in arid and high-temperature environments.
The innovative use of metal/metal oxide as the reference electrode enables miniaturization by eliminating the reference gas and sealing the reference electrode. The combination of interdigitized electrodes with the unique metal/metal oxide reference electrode permits sensor operation in either potentiometric or amperometric mode, as appropriate. In potentiometric mode, which measures voltage differences between working and reference electrodes in different gases, the voltage differences can be monitored with a voltmeter; however, the sensor itself does not need a power source. In room-temperature testing, the sensor achieved repeatable responses to 21 percent oxygen in nitrogen (using nitrogen as a baseline gas), and also detected oxygen from 7 to 21 percent, making Glenn's breakthrough technology usable for personal health monitoring as well as fire detection, fuel-leak detection, and environmental monitoring.

Miniaturized Laser Heterodyne Radiometer
This instrument uses a variation of laser heterodyne radiometer (LHR) to measure the concentration of trace gases in the atmosphere by measuring their absorption of sunlight in the infrared. Each absorption signal is mixed with laser light (the local oscillator) at a near-by frequency in a fast photoreceiver. The resulting beat signal is sensitive to changes in absorption, and located at an easier-to-process RF frequency. By separating the signal into a RF filter bank, trace gas concentrations can be found as a function of altitude.

Portable Medical Diagnosis Instrument
The technology utilizes four cutting-edge sensor technologies to enable minimally- or non-invasive analysis of various biological samples, including saliva, breath, and blood. The combination of technologies and sample pathways have unique advantages that collectively provides a powerful analytical capability. The four key technology components include the following: (1) the carbon nanotube (CNT) array designed for the detection of volatile molecules in exhaled breath; (2) a breath condenser surface to isolate nonvolatile breath compounds in exhaled breath; (3) the miniaturized differential mobility spectrometer (DMS) -like device for the detection of volatile and non-volatile molecules in condensed breath and saliva; and (4) the miniaturized circular disk (CD)-based centrifugal microfluidics device that can detect analytes in any liquid sample as well as perform blood cell counts. As an integrated system, the device has two ports for sample entry a mouthpiece for sampling of breath and a port for CD insertion. The breath analysis pathway consists of a CNT array followed by a condenser surface separating liquid and gas phase breath. The exhaled breath condensate is then analyzed via a DMS-like device and the separated gas breath can be analyzed by both CNT sensor array again and by DMS detectors.

Carbon Dioxide Gas Sensors
Current bulk or thick film solid electrolyte CO2 sensors are expensive, difficult to batch fabricate, and large in size. In contrast, this new amperometric, solid-state, oxide-based electrolyte CO2 microsensor is affordable, easy to fabricate, and is so small that it could easily be integrated onto a substrate the size of a postage stamp.
The basic composition of the sensor is identical to a previously designed NASA Glenn technology in which a solid electrolyte of Na3Zr2Si2PO12 is deposited between interdigitated electrodes on an alumina substrate and is covered by Na2CO3/BaCO3. Unlike its predecessor, however, this innovation includes an additional layer of nanocrystalline SnO2 sol gel, an electron donor type (N-type) semiconductor, on top of the Na2CO3/BaCO3 . This new layer provides a greater number of electrons for reduction reaction at the working electrode to detect CO2. As a result, overall performance is enhanced, and this new state-of-the-art sensor has the ability to operate at temperatures as low as 375°C. This low temperature capability significantly decreases the amount of power required to operate the sensor, opening the door to a multitude of new applications that were previously unattainable.