Liquid Sorbent Carbon Dioxide Removal System
mechanical and fluid systems
Liquid Sorbent Carbon Dioxide Removal System (MSC-TOPS-84)
Using a 3D-Printed Capillary Microchannel Contactor
Overview
Innovators at the NASA Johnson Space Center (JSC), in collaboration with Jacobs Technology, and IRPI, have developed a reliable, efficient, and cost-effective carbon dioxide (CO2) removal and dehumidification system. The new system is designed for ventilation applications and utilizes a gentle, passive, and direct air/liquid contactor. The contactor is composed of a bifurcating manifold with 3D printed corrugated walls, that contain capillary channels onto which thin films of liquid sorbent are deployed. The liquid is held in place by surface tension and capillary forces. As the liquid is exposed to the air, it absorbs carbon dioxide and humidity from the environment. NASA's new CO2 removal system has significant advantages over current CO2 scrubbers. For example, the new system eliminates the need for large blowers and compressors that force air at high velocities through adsorption-based systems using solid sorbents.
The Technology
NASA's Liquid Sorbent Carbon Dioxide Removal System was designed as an alternative to the current CO2 removal technology used on the International Space Station (ISS), which uses solid zeolite media that is prone to dusting, has a low absorption capacity, and requires high regeneration temperatures and frequent maintenance. Motivated by CO2 removal systems on submarines, NASA innovators began investigating the use of liquid sorbents. Liquid sorbents have a capacity four times greater than solid zeolites, require low regeneration temperature, and need fewer unreliable moving mechanical parts than solid based systems. While submarine CO2 scrubbers spray an adsorbing chemical directly into the air stream and allow the liquid to settle, NASA's new system uses a capillary driven 3D printed microchannel direct air/liquid contactor in a closed loop system. The Liquid Sorbent Carbon Dioxide Removal System is robust and reliable, while being low in weight, volume, and power requirements. The system is capable of reaching equilibrium when the liquid sorbent surface is being regenerated at a rate equal to the rate of absorption into the liquid.
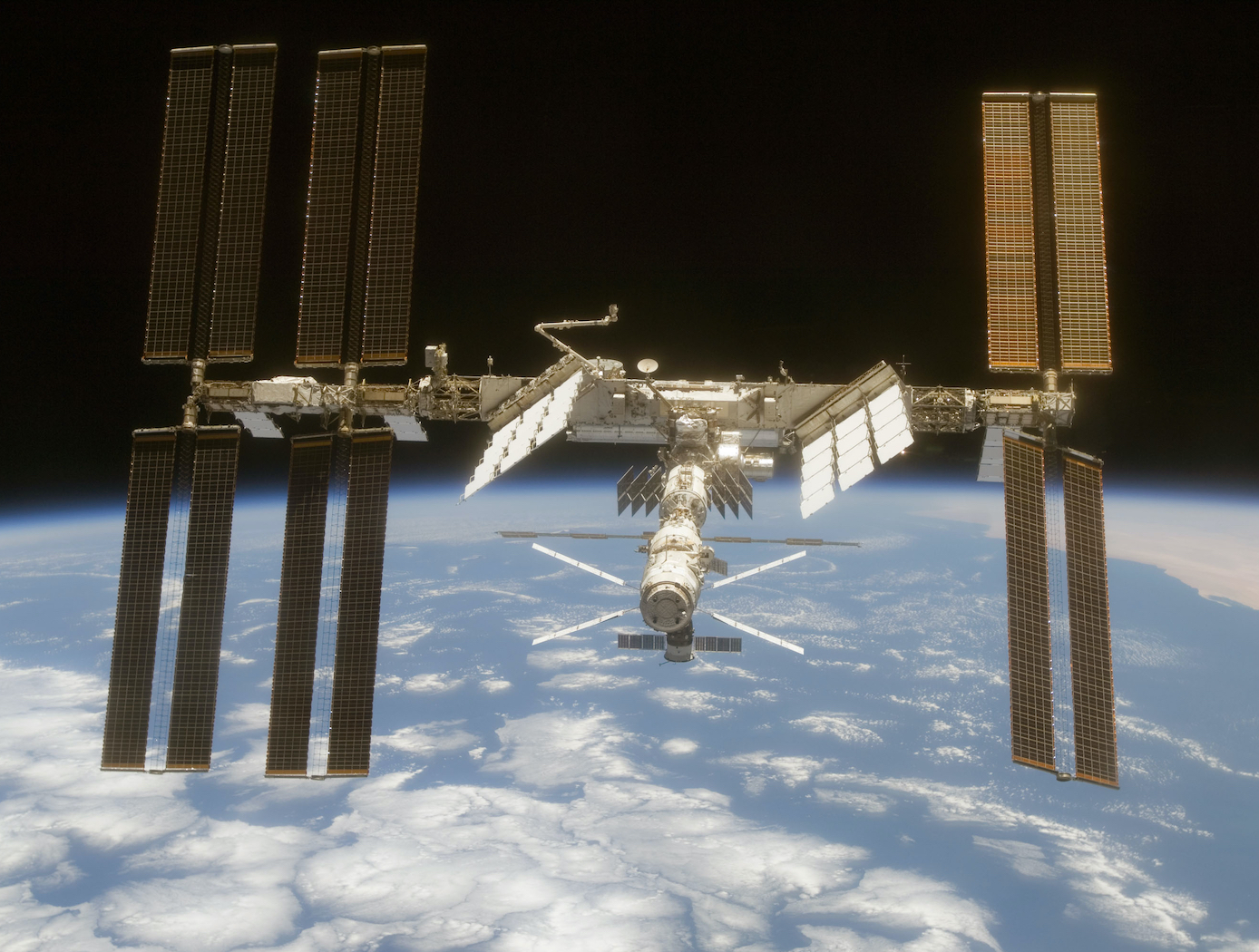

Benefits
- Enables simplified system integration: Does not require large blowers and compressors that need to force air at high velocities through typical CO2 removal systems
- Offers a reliable, efficient design: Requires fewer moving mechanical parts than solid based systems and offers low weight, volume, and power requirements
- Presents improvements over current technologies: Liquid sorbents have favorable capacity, up to four times greater than current solid zeolites
- Facilitates simple regeneration techniques: Liquid sorbents have low regeneration temperatures, so the materials do not need to be heated to extreme temperature
Applications
- Aerospace
- Agriculture: Controlled Atmosphere Storage
- Automotive: Recirculating Air Conditioning Systems
- Chemical Manufacturing
- Commercial Space Flight
- Consumer Goods: Rebreathers for Scuba Diving
- First Responders: Rebreather Systems
- Marine: Submarines and Submersible Craft
Technology Details
mechanical and fluid systems
MSC-TOPS-84
MSC-26442-1
"Liquid Behavior through a Capillary Microchannel Contactor in a Reduced Gravity Aircraft," Tanya Rogers, John Graf & Julia Worrell, 07/16/2017,
https://ttu-ir.tdl.org/handle/2346/72942
https://ttu-ir.tdl.org/handle/2346/72942
Tags:
|
Similar Results

Air Revitalization for Vacuum Environments
The NASA life support system uses a regenerable vacuum swing adsorption process, known as Sorbent-Based Air Revitalization (SBAR), to separate water and carbon dioxide for disposal. The SBAR system is an adsorbent-based swing bed system that has been optimized to provide both humidity and carbon dioxide control for a spacecraft cabin atmosphere.
The system comprises composite silica gel and zeolite-packed beds for adsorption and a bypass system for flow control. Under normal operating conditions, the disposal system would require a high-quality vacuum environment to operate. Improvements to the SBAR system include an enhanced inherent capacitance that extends the operation time within a non-vacuum environment for up to 4.5 hours. Flight time can be further expanded with multiple SBAR systems to allow for system regeneration. By scheduling periodic thermal regenerations—nominally during sleep periods—the SBAR technology may be suitable for missions of unlimited duration.
Cryogenic Oxygen Storage Modules COSM
The COSM employs NASA's Cryogenic Flux Capacitor core to store liquid oxygen (at 90 K) in silica aerogel material at ambient pressure, and then discharges cold oxygen gas into an in-line flow loop in response to heat input. If the composition of the incoming effluent stream contains gases with condensation or freezing points above the 90 K oxygen storage temperature--such as carbon dioxide or water vapor--these gasses can be removed from the stream as it moves through the COSM. The current COSM is sized to be wearable on the person but can be easily scaled to much larger sizes and various geometries.
COSM is designed with a long "cold path" which provides for greater residence times which increase the probability that condensable/freezable gases will be trapped in the COSM. Also, the longer the cold path, the longer the time a COSM can be used prior to the oxygen being depleted and the scrubbed gasses liberated. Two COSM geometries have been designed, built, and tested-a round spiral and a prismatic serpentine--to achieve long cold paths, and intrinsic vapor cooling to manage heat loads.

Cryogenic Flux Capacitor
Storage and transfer of fluid commodities such as oxygen, hydrogen, natural gas, nitrogen, argon, etc. is an absolute necessity in virtually every industry on Earth. These fluids are typically contained in one of two ways; as low pressure, cryogenic liquids, or as a high pressure gases. Energy storage is not useful unless the energy can be practically obtained ("un-stored") as needed. Here the goal is to store as many fluid molecules as possible in the smallest, lightest weight volume possible; and to supply ("un-store") those molecules on demand as needed in the end-use application. The CFC concept addresses this dual storage/usage problem with an elegant charging/discharging design approach.
The CFC's packaging is ingeniously designed, tightly packing aerogel composite materials within a container allows for a greater amount of storage media to be packed densely and strategically. An integrated conductive membrane also acts as a highly effective heat exchanger that easily distributes heat through the entire container to discharge the CFC quickly, it can also be interfaced to a cooling source for convenient system charging; this feature also allows the fluid to easily saturate the container for fast charging. Additionally, the unit can be charged either with cryogenic liquid or from an ambient temperature gas supply, depending on the desired manner of refrigeration. Finally, the heater integration system offers two promising methods, both of which have been fabricated and tested, to evenly distribute heat throughout the entire core, both axially and radially.
NASA engineers also applied the CFC to a Cryogenic Oxygen Storage Module to store oxygen in solid-state form and deliver it as a gas to an end-use environmental control and/or life support system. The Module can scrub out nuisance or containment gases such as carbon dioxide and/or water vapor in conjunction with supplying oxygen, forming a synergistic system when used in a closed-loop application. The combination of these capabilities to work simultaneously may allow for reduced system volume, mass, complexity, and cost of a breathing device.

Filtering Molecules with Nanotube Technology
This water filtration innovation is an acoustically driven molecular sieve embedded with small-diameter carbon nanotubes. First, water enters the device and contacts the filter matrix, which can be made of polymer, ceramic, or metallic compounds. Carbon nanotubes within the matrix allow only water molecules to pass through, leaving behind any larger molecules and contaminants. The unique aspect of the technology is its use of acoustics to help drive water through the filter.
An oscillator circuit attached to the filter matrix propagates acoustic vibration, further causing water molecules to de-bond and move through the filter. This use of acoustics also eliminates dependence on gravity (and thus filter orientation) to move water through the device. When water exiting the system diminishes to a pre-determined set point, a cleaning cycle is triggered to clear the sediment from the inlet of the filter, reestablishing the standard system flow rate. Unlike other filtration systems, flushing of the filter system is not required. The combination of acoustics and small-diameter carbon nanotubes in this innovation make it an effective and efficient means of producing contaminant-free, clean water.

Carbonated Cement for Production of Concrete with Improved Properties
The NASA cement innovation describes a method to make solid carbon material from CO2 captured during the cement-making process, and for using that carbon material in the mixture to improve cement properties. Doing so provides a direct use for the captured CO2, eliminating any CO2 storage/disposal issues and providing an improved cement product.
The innovation employs a chemical reaction, known as the Bosch process, which uses hydrogen gas and catalysis to reduce the CO2 to solid carbon and water. Cement manufacturing is uniquely suited to the use of the Bosch process. Cement manufacturing requires high temperatures, and harnessing this excess heat limits the total energy required to maintain a Bosch process at a cement plant. Also, cement contains iron, a metal shown to be an exceptional catalyst for the Bosch process. Thus, the cement product itself can be used as the catalyst for the reaction, also serving as a carbon sink. This eliminates any requirements for the storage or disposal of the waste carbon captured from CO2 emissions.
Test evaluations at the bench scale have provided encouraging indications of enhanced mechanical properties for the carbon-containing cement materials. In particular, the findings suggest that the carbon in the concrete might delay the environmental breakdown of concrete due to the blocking effect of the carbon on harmful ions (e.g., chlorine).