Ultrasonic Stir Welding
manufacturing
Ultrasonic Stir Welding (TOP8-95)
A new solid-state weld process for better weld quality and longer tool life
Overview
NASA Marshall Space Flight Center (MSFC) developed Ultrasonic Stir Welding (USW) to join large pieces of very high-strength metals such as titanium and Inconel. USW, a solid-state weld process, improves current thermal stir welding processes by adding high-power ultrasonic (HPU) energy at 20 kHz frequency. The addition of ultrasonic energy significantly reduces axial, frictional, and shear forces; increases travel rates; and reduces wear on the stir rod, which results in extended stir rod life. The USW process decouples the heating, stirring, and forging elements found in the friction stir welding process allowing for independent control of each process element and, ultimately, greater process control and repeatability. Because of the independent control of USW process elements, closed-loop temperature control can be integrated into the system so that a constant weld nugget temperature can be maintained during welding.
The Technology
Ultrasonic Stir Welding is a solid state stir welding process, meaning that the weld work piece does not melt during the welding process. The process uses a stir rod to stir the plasticized abutting surfaces of two pieces of metallic alloy that forms the weld joint. Heating is done using a specially designed induction coil. The control system has the capability to pulse the high-power ultrasonic (HPU) energy of the stir rod on and off at different rates from 1-second pulses to 60-millisecond pulses. This pulsing capability allows the stir rod to act as a mechanical device (moving and stirring plasticized nugget material) when the HPU energy is off, and allowing the energized stir rod to transfer HPU energy into the weld nugget (to reduce forces, increase stir rod life, etc.) when the HPU energy is on. The process can be used to join high-melting-temperature alloys such as titanium, Inconel, and steel.
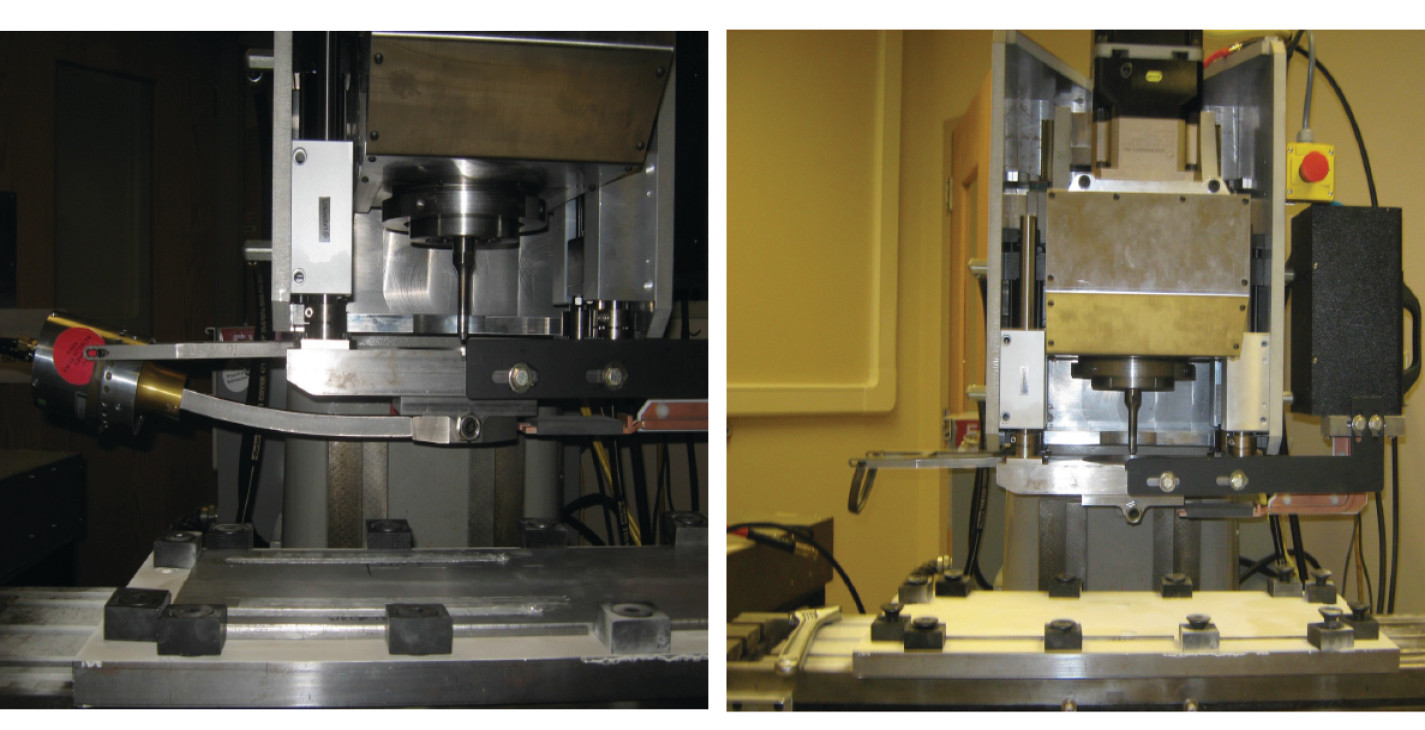
Benefits
- Improved weld properties
- Increased tool life (stir rods, bushings, containment plates)
- Automated closed-loop feedback control
- Potential for integration into robotic welders
- Reduced axial and shear consolidation forces
- Potential for handheld version
- Reduced friction without lubricants
Applications
- Aerospace - hardware for severe environments, launch vehicles, aircraft
- Automotive - pistons, struts, vehicle structure
- Marine - shipbuilding, platforms
- Civil - bridges, trains, pressure vehicles
Tags:
|
Similar Results

Internal Friction Reduction (IFR) Tool
During SR-FSW, the wicking of substrate material into the gap between the crown shoulder and pin can lead to pinch load shifting–an increasing difference between the applied pin load and actual pinch load. NASA's IFR Tool mitigates pinch load shifting, enabling a more robust SR-FSW process due to better agreement between the applied pin load and the actual pinch load.
As pinch loads are not dynamically measured during welding, NASA has relied on defect rates and pin tool ejection loads to demonstrate the value of the IFR Tool. Large-scale welds were performed with both the IFR Tool and a standard pin tool. Weld inspections found a 90% reduction in defect generation rate for the IFR tool, and the first defect in the standard pin tool sample occurred at 300" compared to at 900" in the IFR Tool sample. There was also a drastic improvement in mechanical properties variations along the weld.
In another study, a series of 27.5' barrel welds were performed with both the IFR Tool and a standard pin tool. After welding, ejection forces required to remove the pin tools from their fixtures were measured. The standard pin tool ejection force was 9,400 lbs., nearly 5 times larger than that of the IFR Tool at 2,000 lbs . The large difference suggests that during welding, the standard pin tool would have been less responsive to substrate surface variations than the IFR Tool. Weld inspections supported this theory, as they found a significantly lower defect rate in the IFR Tool samples.

Friction Stir Deposition Innovations
Metal additive manufacturing may be limited by build volumes (i.e., it can be hard to make large parts), post-processing requirements, and upfront costs to buy capital equipment. The two NASA-developed technologies are add-on tools for FSW systems (reducing costs), do not require a printer or print bed, and produce parts with high quality surface finishes.
The C-FSD attachment includes a non-rotating block through which the C-FSW rotating pin is threaded, and a containment plate to hold the plasticized metal within the system. In this technique, raw metal feedstock is fed into one end of the non-rotating block, is heated and plasticized by the C-FSW pin, and is driven out the other side of the block. The C-FSW pin is used to join the new material to the pre-existing layer.
The B-FSD tool uses a dual-shoulder design to print outward from the edge of the base panel. The B-FSD process uses the same feed system as the C-FSD, but utilizes the bobbin/SR-FSW pin's dual shoulders (i.e., containing the metal on both the top and bottom) enabling more complex structures to be made, and the ability to print varying thickness depositions in a single pass.
The Additive C-FSD and B-FSD end effector tools are both at technology readiness level (TRL) 4 (component and/or breadboard validation in laboratory environment) and are available for patent licensing.

Conventional friction stir extrusion machine
Typical metal extrusion relies on heating large metal billets and then forcing the heated billet through a dye to extrude the geometry and length of interest. These processes require high energy inputs, expensive machinery to heat and manipulate the billets, and the length of the final part is limited by billet size. Thus, new ways to cost effectively and efficiently produce extruded parts are needed.
The C-FSE machine developed by NASA encompasses a non-rotating extrusion block and a rotating pin that extends through the chamber. The extrusion block has a close tolerance fit to the rotating pin to prevent material from escaping from the ends of the block. Raw metal feedstock is fed into one side of the chamber, the rotating pin interacts with the metal to generate plastic deformation and heat, and the metal is driven out the other side of the extrusion block through a customizable die. As the C-FSE machine does not require pre-heated billets, the extruded parts may be of any desired length. Further, the extrusion machine is modular in nature and may be retrofitted onto an existing FSW system, and the die may be easily replaced for varying extrusion geometries. The C-FSE machine has been prototyped and used to produce freestanding metal parts.
The C-FSE machine is at technology readiness level (TRL) 4 (component and/or breadboard validation in laboratory environment) and is available for patent licensing.

Abnormal Grain Growth Suppression in Aluminum Alloys
Heat treatment of the deformed welds is desirable in order to restore the properties of the alloy negatively affected in the weld region. In these alloys, abnormal grain growth frequently occurs in friction stir welds during solution heat treatment, and is known to degrade key materials properties, such as strength, ductility and toughness. The innovation of inserting an intermediate annealing step covered here reduces abnormal grain growth during post-welding heat treatment, thereby allowing optimum mechanical properties. This is important where Al-Li alloys (and other heat treatable alloys) are friction stir welded followed by deformation processing and high performance, high reliability structural components are required for aerospace vehicles.

Additively Manufactured Oscillating Heat Pipe for High Performance Cooling in High Temperature Applications
The advent of additive manufacturing makes available new and innovative integrated thermal management systems, including integrating an oscillating Heat Pipe (OHP) into the leading edge of a hypersonic vehicle for rapid dissipation of large quantities of heat. OHPs have interconnected capillary channels filled with a working fluid that forms a train of liquid plugs and vapor bubbles to facilitate rapid heat transfer. Multiple additive manufacturing techniques may be used, including powder bed fusion, binder jetting, metal material extrusion, directed energy deposit, sheet lamination, ultrasonic, and electrochemical techniques. These high performance OHPs can be made with materials such as Refractory High Entropy Alloys (RHEAs) that can withstand high temperature applications. The structure of the OHP can be integrated into the constructed leading edge. The benefits include a heat transport capacity of 10 to 100 times greater than before. Integrated OHPs avoid the bends or welds in traditional heat pipes, especially at the locations where the highest thermal stresses might cause thermal-structural failure of a leading edge. Alternating the diameters of the OHP channels alleviate start-up issues typically found in liquid metal oscillating heat pipe designs in high temperature applications by aiding in the instigation of a circulating flow due to multiple forces acting upon the working fluid.