Search
PATENT PORTFOLIO
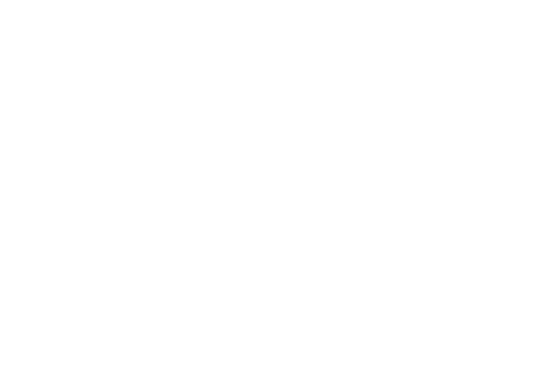
Communications
NASA's portfolio of communications technology represents a diverse range of innovative solutions for transmitting and receiving information in a variety of environments. Whether it's enabling communication between spacecraft and mission control or providing high-speed internet access on Earth, these patents showcase the many ways in which NASA is shaping the future of communication.
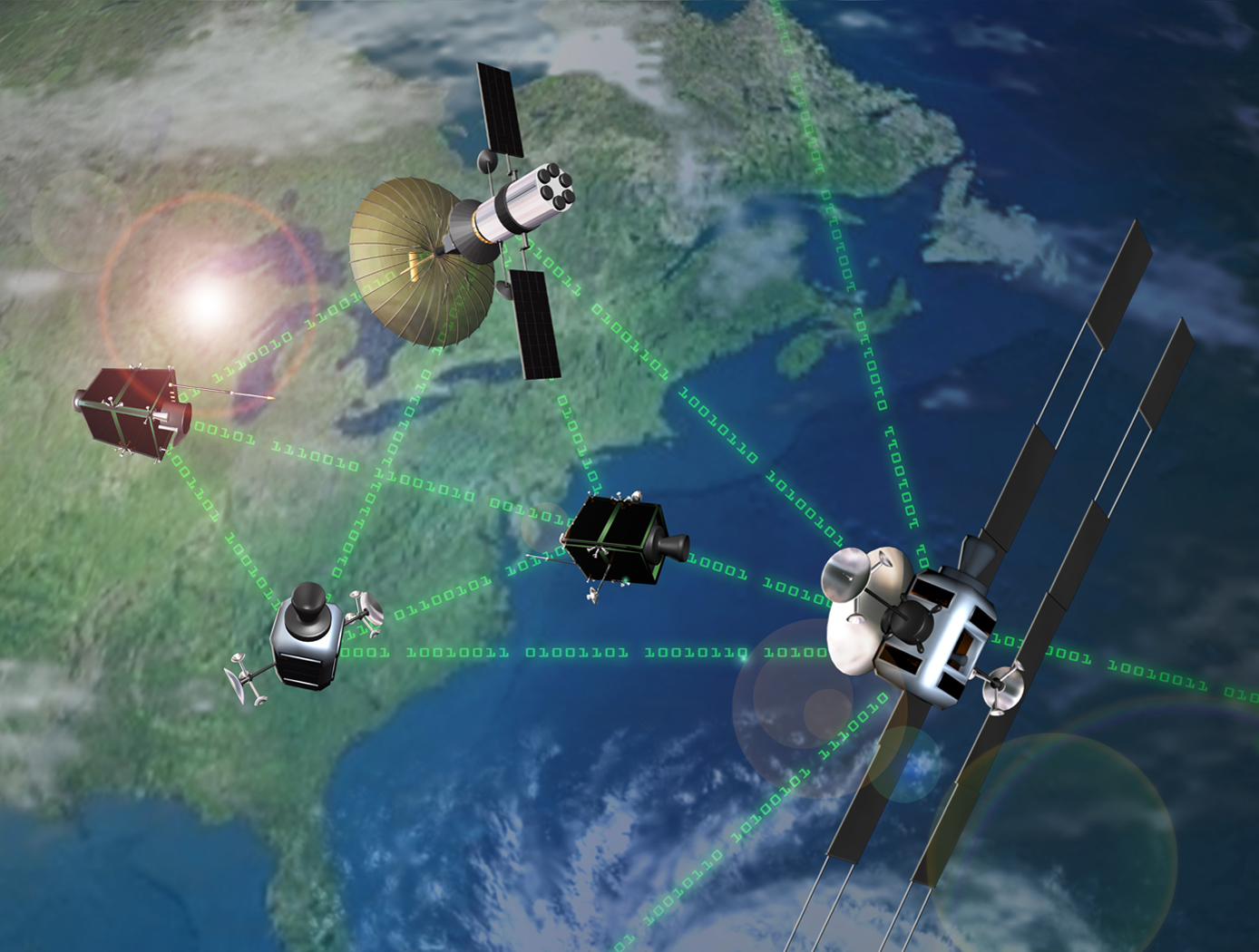
Heterogeneous Spacecraft Networks
Heterogeneous Spacecraft Networks address an emerging need, namely, the ability of satellites and other space-based assets to freely communicate with each other. While it appears that there has been no significant effort to date to address the application, emergence of such a solution is inevitable, given the rapidly-growing deployments of small satellites. These assets need to be able to communicate with each other and with global participants. Extending established global wireless network platforms like Wi-Fi and ZigBee to space-based assets will allow different satellite clusters to assist each other. For example, one cluster could provide images of the earths surface when another cluster is with out visibility at the needed time and location. More importantly, use of such common platforms will enable collaboration among individuals, institutions, and countries, each with limited assets of its own. Thus, allowing the incorporation of space-based assets into commercial wireless networks, and extending commercial communications into low Earth orbit satellites, access to satellite data will become ubiquitous.Similarly, some global networks will also benefit from the ability of a variety of nodes of different types to communicate with each other. One instance is in the emerging Internet of Things (IoT), where an enormous number of smart objects work together to provide customized solutions.
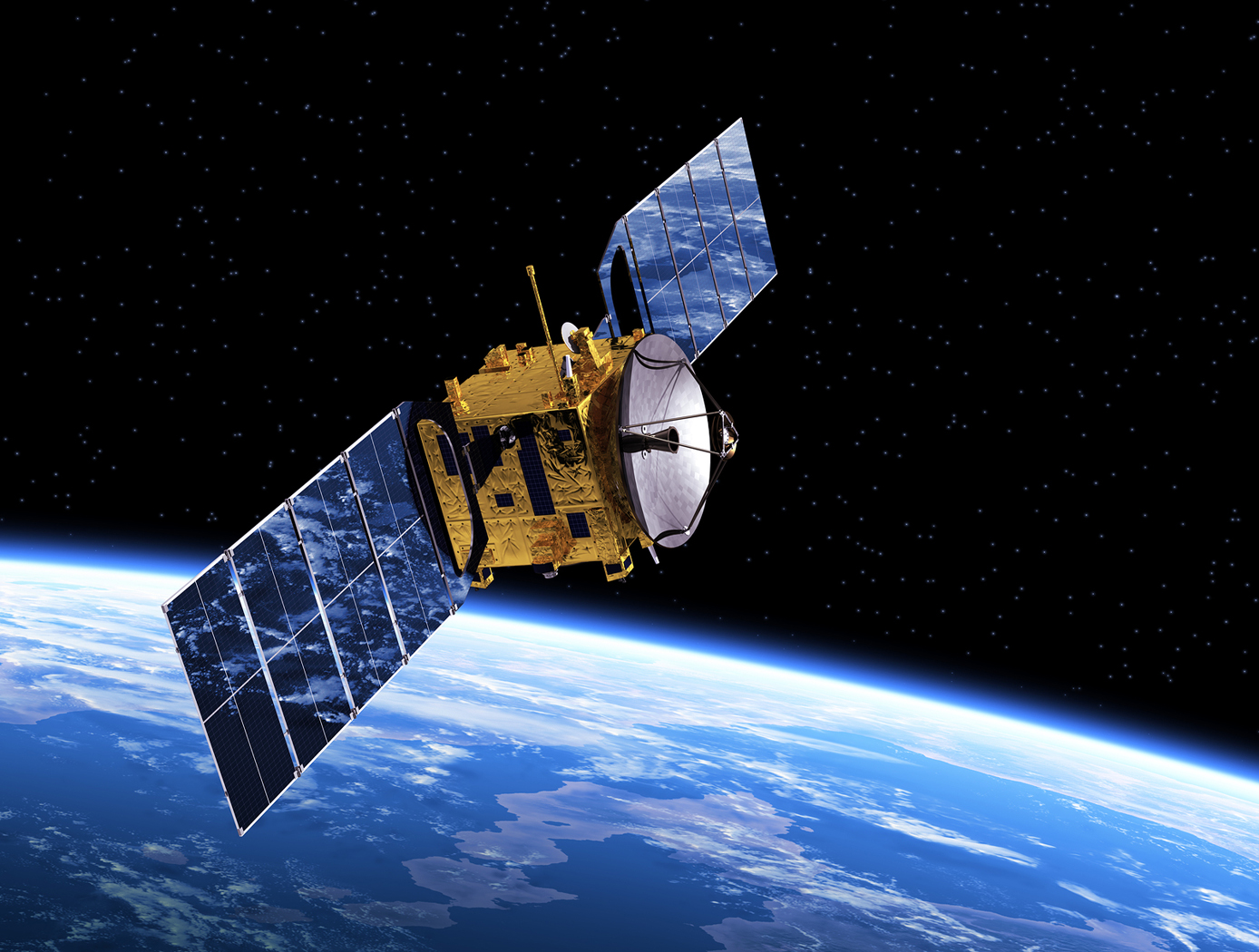
Space Optical Communications Using Laser Beams
This invention provides a new method for optical data transmissions from satellites using laser arrays for laser beam pointing. The system is simple, static, compact, and provides accurate pointing, acquisition, and tracking (PAT). It combines a lens system and a vertical-cavity surface-emitting laser VCSEL)/Photodetector Array, both mature technologies, in a novel way for PAT. It can improve the PAT system's size, weight, and power (SWaP) in comparison to current systems. Preliminary analysis indicates that this system is applicable to transmissions between satellites in low-Earth orbit (LEO) and ground terminals. Computer simulations using this design have been made for the application of this innovation to a CubeSat in LEO. The computer simulations included modeling the laser source and diffraction effects due to wave optics. The pointing used a diffraction limited lens system and a VCSEL array. These capabilities make it possible to model laser beam propagation over long space communication distances. Laser beam pointing is very challenging for LEO, including science missions. Current architectures use dynamical systems, (i.e., moving parts, e.g., fast-steering mirrors (FSM), and/or gimbals) to turn the laser to point to the ground terminal, and some use vibration isolation platforms as well. This static system has the potential to replace the current dynamic systems and vibration isolation platforms, dependent on studies for the particular application. For these electro-optical systems, reaction times to pointing changes and vibrations are on the nanosecond time scale, much faster than those for mechanical systems. For LEO terminals, slew rates are not a concern with this new system.
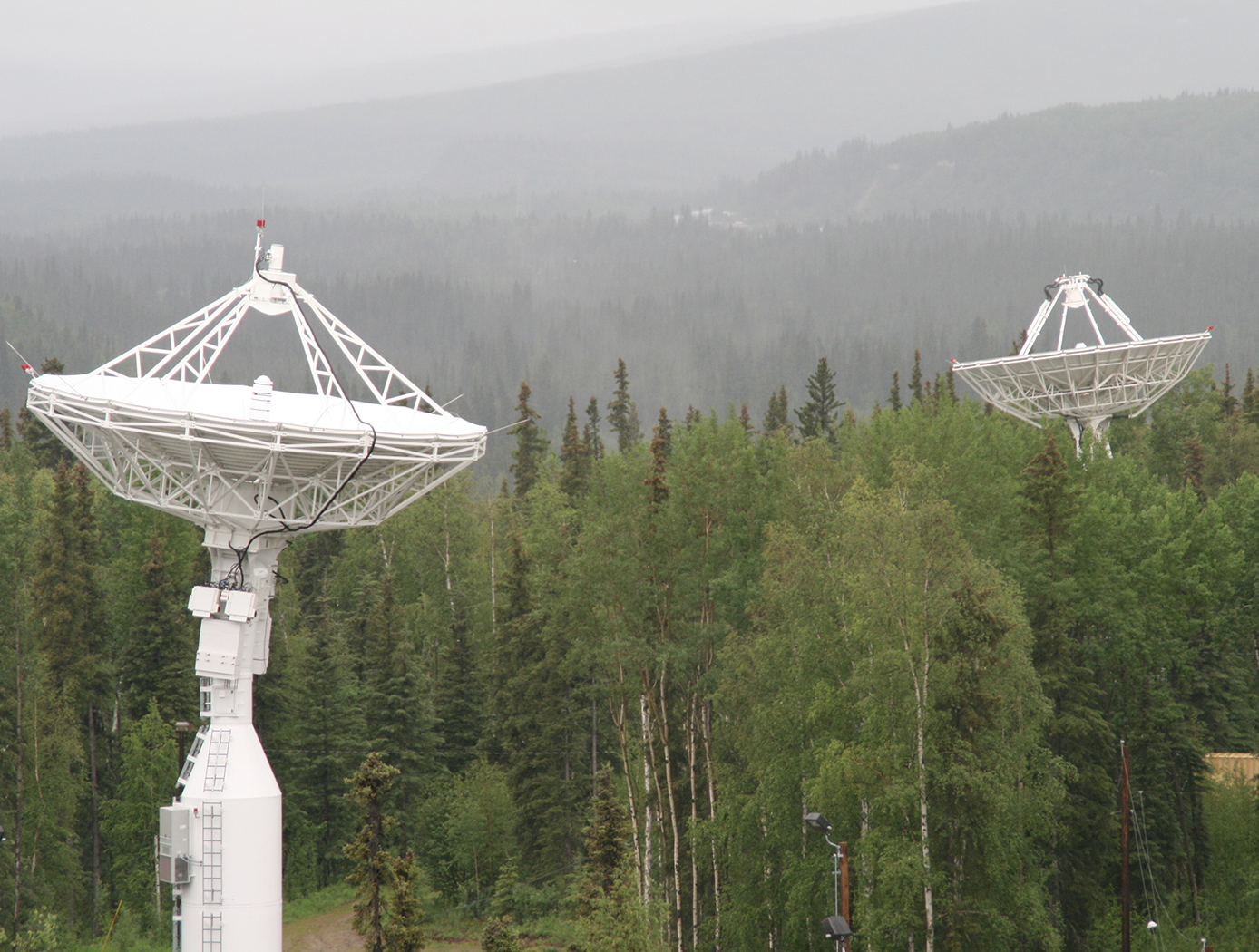
Signal Combiner for Wideband Communication
Through low-loss signal combination, Glenn is leading the way to optimize radio transmission remotely during self-checking routines. Glenn's signal combiner offers a simple method to minimize signal loss significantly when combining two signals. Using conventional combiners in bit-error-rate testing results in a loss of 3 to 4 dB per band, and with a directional coupler the secondary signal experiences losses of 10 dB or more. Moreover, during signal measurements, the additional components must be placed and later removed to prevent any impact to the measurement, making for a cumbersome process. Glenn's solution is to combine the primary and secondary signals in the frequency domain through the use of a frequency division diplexer/multiplexer in combination with a wideband ADC. The multiplexer selects one or more bands in the frequency domain, and the ADC performs a non-linear conversion to digital domain by folding out-of-band signals in with the primary signal. NASA makes use of subsampling a given band within the ADC bandwidth to fold it into another band of interest, effectively frequency-shifting them to a common frequency bandwidth.
Glenn's breakthrough method has two significant advantages over the conventional use of a power combiner or directional coupler in bit-error-rate testing: 1) it combines signal and noise (secondary signal) with very low loss, and 2) it enables the selection of the desired signal-to-noise ratio with no need for the later cumbersome removal of components. This streamlined process allows for invaluable in-situ or installed measurement. Glenn's novel technology has great potential for satellite, telecommunications, and wireless industries, especially with respect to equipment testing, measurement, calibration, and check-out.
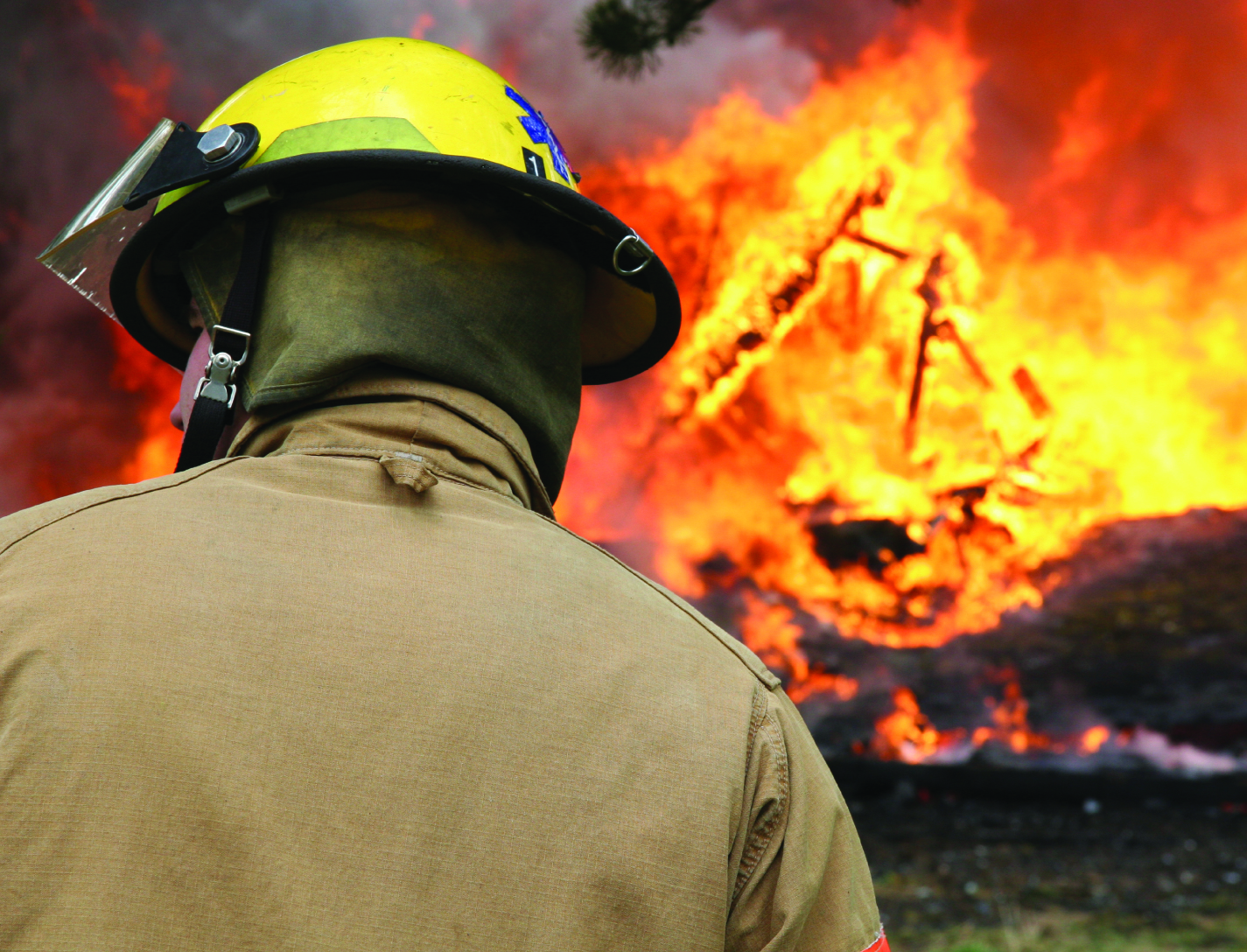
Real-Time Tracking System
The innovation builds upon conventional UWB hardware by incorporating tracking methodology and algorithms in addition to external amplifiers for signal boost. The tracking methodology is a triangulation calculation consisting of Angle of Arrival (AOA) and Time Difference of Arrival (TDOA) using a cross-correlation peak detection method. By directly estimating TDOA information from UWB pulses, the method achieves the high temporal resolution (on the order of picoseconds) needed to measure AOA with extreme precision. The system uses a PC to synchronize and process data in real time from two receivers, or clusters, to display the position of the transmitter-equipped person or object. The interface software enables the PC to access the two data sets simultaneously through separate sockets. In the data collection process, data segments from each receiver are interleaved with those from the other receiver in chronological order of collection. Within the PC, the data segments are stored in a separate buffer; therefore, the contents of the buffers are representations of the same UWB pulse waveform arriving at the two receivers at approximately the same time. This data synchronization provides the separate and simultaneous collection of waveform data that the tracking algorithm requires for accurate real-time tracking.
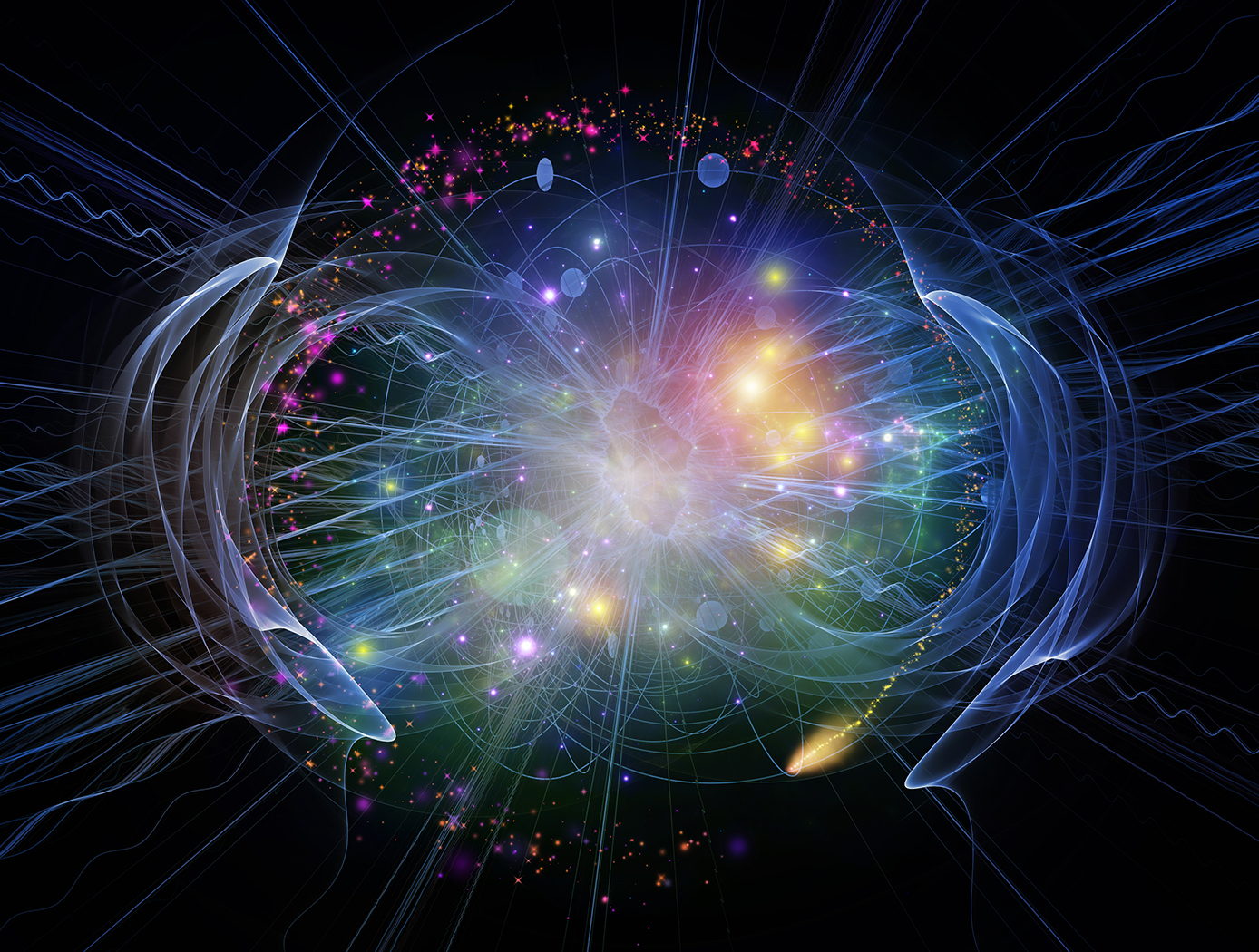
Secure Optical Quantum Communications
In the prior art, the systems that produced photon pairs took up a great deal of space on a laboratory table, weighed several hundred pounds, consumed tens of kilowatts of electrical power, and required cooling water. These limitations greatly restricted the utility of quantum communications systems, which rely on these photon pairs. To address this issue, Glenn's innovators developed a novel system that uses a laser source, a pair of nonlinear crystals in optical contact with each other, and a fiber coupling point configured to receive a pair of single mode fibers. Pairs of polarization-entangled photons are produced through spontaneous parametric down conversion of the laser beam and provided to the fiber coupling point. The optical signal is coded at the transmitter by modulating the inter-beam delay time between pairs of entangled photons. The inter-beam delay will determine whether the photon pairs are absorbed by a fluorescer in the receiver. When absorbed, the photon pairs cause a fluorescent optical emission that a photon detector identifies. One advantage of this system is that it eliminates the need for a coincidence counter to realize the entanglement-based secure optical communications, because the absorber acts as a coincidence counter for entangled photon pairs. In addition, this modulation spectroscopy technique is ultra-secure since the delay times are very short (femtoseconds) and unresolvable by conventional photon detectors. Finally, the system uses solid-state, monolithic construction that allows for cost-effective batch-manufacturing techniques. This technology represents a significant breakthrough in the fields of communications, optics, cryptography, and surveillance.
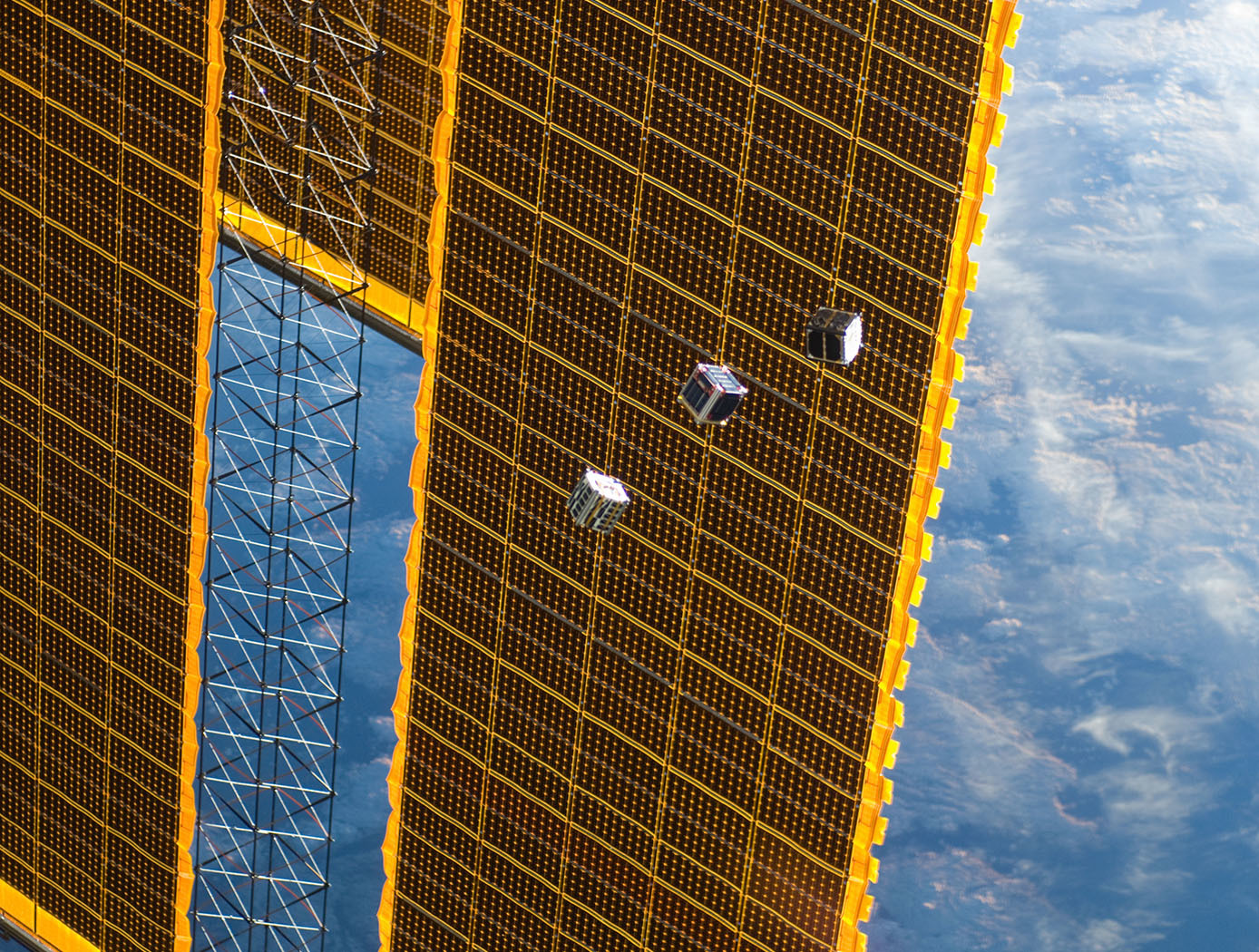
Tunable Multi-Tone, Multi-Band, High-Frequency Synthesizer
Glenn's revolutionary new multi-tone, high-frequency synthesizer can enable a major upgrade in the design of high data rate, wide-band satellite communications links, in addition to the study of atmospheric effects. Conventional single-frequency beacon transmitters have a major limitation: they must assume that atmospheric attenuation and group delay effects are constant at all frequencies across the band of interest. Glenn's synthesizer overcomes this limitation by enabling measurements to be made at multiple frequencies across the entire multi-GHz wide frequency, providing much more accurate and actionable readings.
This novel synthesizer consists of a solid-state frequency comb or harmonic generator that uses step-recovery semiconductor diodes to generate a broad range of evenly spaced harmonic frequencies, which are coherent and tunable over a wide frequency range. These harmonics are then filtered by a tunable bandpass filter and amplified to the necessary power level by a tunable millimeter-wave power amplifier. Next, the amplified signals are transmitted as beacon signals from a satellite to a ground receiving station. By measuring the relative signal strength and phase at ground sites the atmospheric induced effects can be determined, enabling scientists to gather essential climate data on hurricanes and climate change. In addition, the synthesizer can serve as a wideband source in place of a satellite transponder, making it easier to downlink high volumes of collected data to the scientific community. Glenn's synthesizer enables a beacon transmitter that, from the economical CubeSat platform, offers simultaneous, fast, and more accurate wideband transmission from space through the Earth's atmosphere than has ever been possible before.
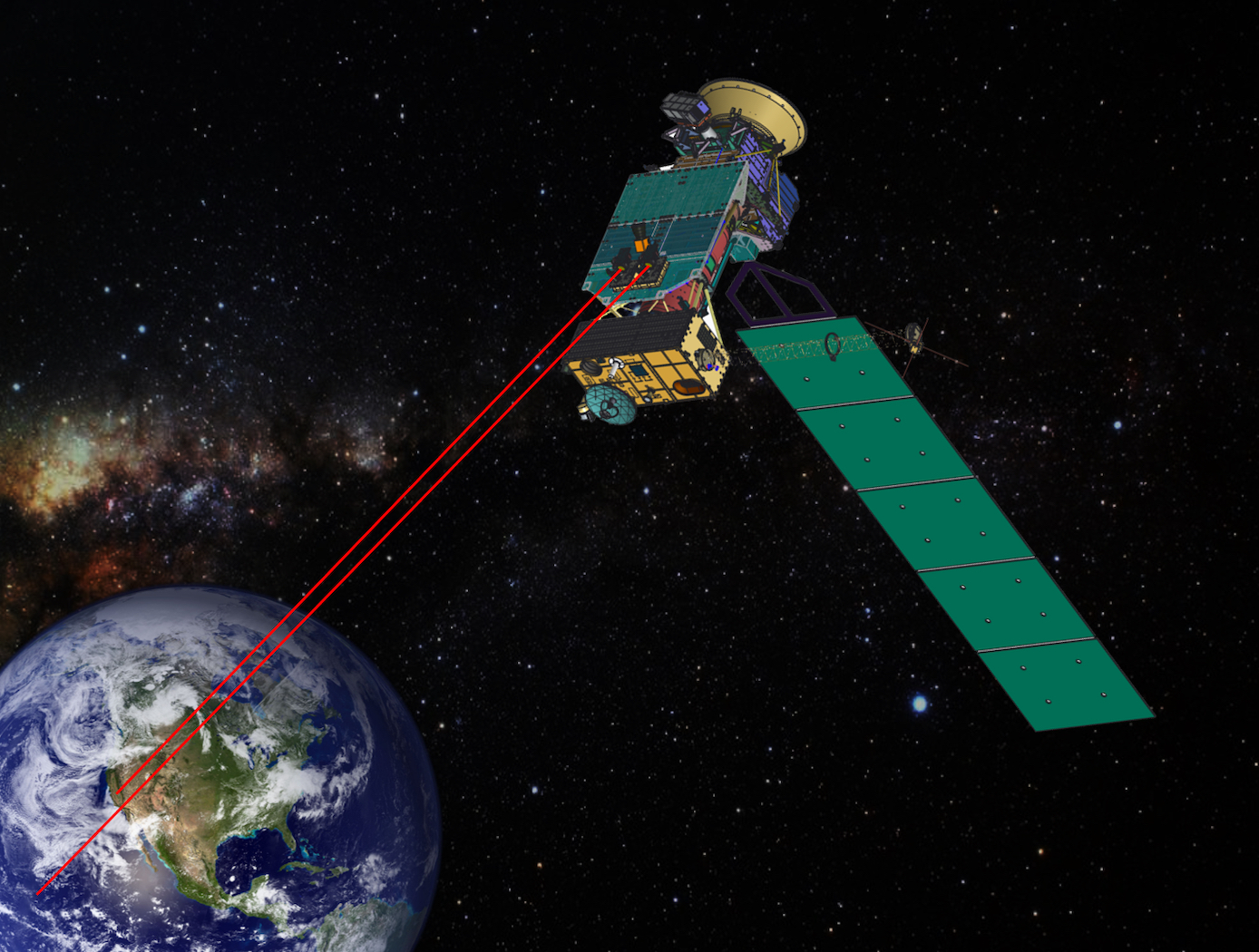
Cascaded Offset Optical Modulator
A unique challenge in the development of a deep space optical SDR transmitter is the optimization of the ER. For a Mars to Earth optical link, an ER of greater than 33 dB may be necessary. A high ER, however, can be difficult to achieve at the low Pulse Position Modulation (PPM) orders and narrow slot widths required for high data rates. The Cascaded Offset Optical Modulator architecture addresses this difficulty by reducing the width of the PPM pulse within the optical modulation subsystem, which relieves the SDR of the high signal quality requirements imposed by the use of an MZM. With the addition of a second MZM and a variable time delay, all of the non-idealities in the electrical signal can be compensated by slightly offsetting the modulation of the laser. The pulse output is only at maximum intensity during the overlap of the two MZMs. The width of the output pulse is effectively reduced by the offset between MZMs. Measurement and analysis of the system displayed, for a 1 nanosecond pulse width, extinction ratios of of 32.5 dB, 39.1 dB, 41.6 dB, 43.3 dB, 45.8 dB, and 48.2 dB for PPM orders of 4, 16, 32, 64, 128, and 256, respectively. This approach is not limited to deep space optical communications, but can be applied to any optical transmission system that requires high fidelity binary pulses without a complex component. The system could be used as a drop-in upgrade to many existing optical transmitters, not only in free space, but also in fiber. The system could also be implemented in different ways. With an increase in ER, the engineer has the choice of using the excess ER for channel capacity, or simplifying other parts of the system. The extra ER could be traded for reduced laser power, elimination of optical amplifiers, or decreased system complexity and efficiency.

Multi-and Wide-Band Single-Feed Patch Antenna
NASA's patch antenna technology exhibits higher operational bandwidth (on the order of 20%) than typical patch antennas (less than 10%) and can operate across integer-multiple frequency bands (e.g. S/X, C/X, S/C). Testing of the antenna design has demonstrated > 6dB of gain on both S and X bands (boresight), with an axial ratio of < 6dB and voltage standing wave ratio (VSWR) < 3:1 throughout the entire near-Earth network (NEN) operating bands (22.4GHz and 88.4GHz) with hemispherical coverage. The patch size is on the order of 10 x 10 cm and with associated electronics, is about 1 cm in height.
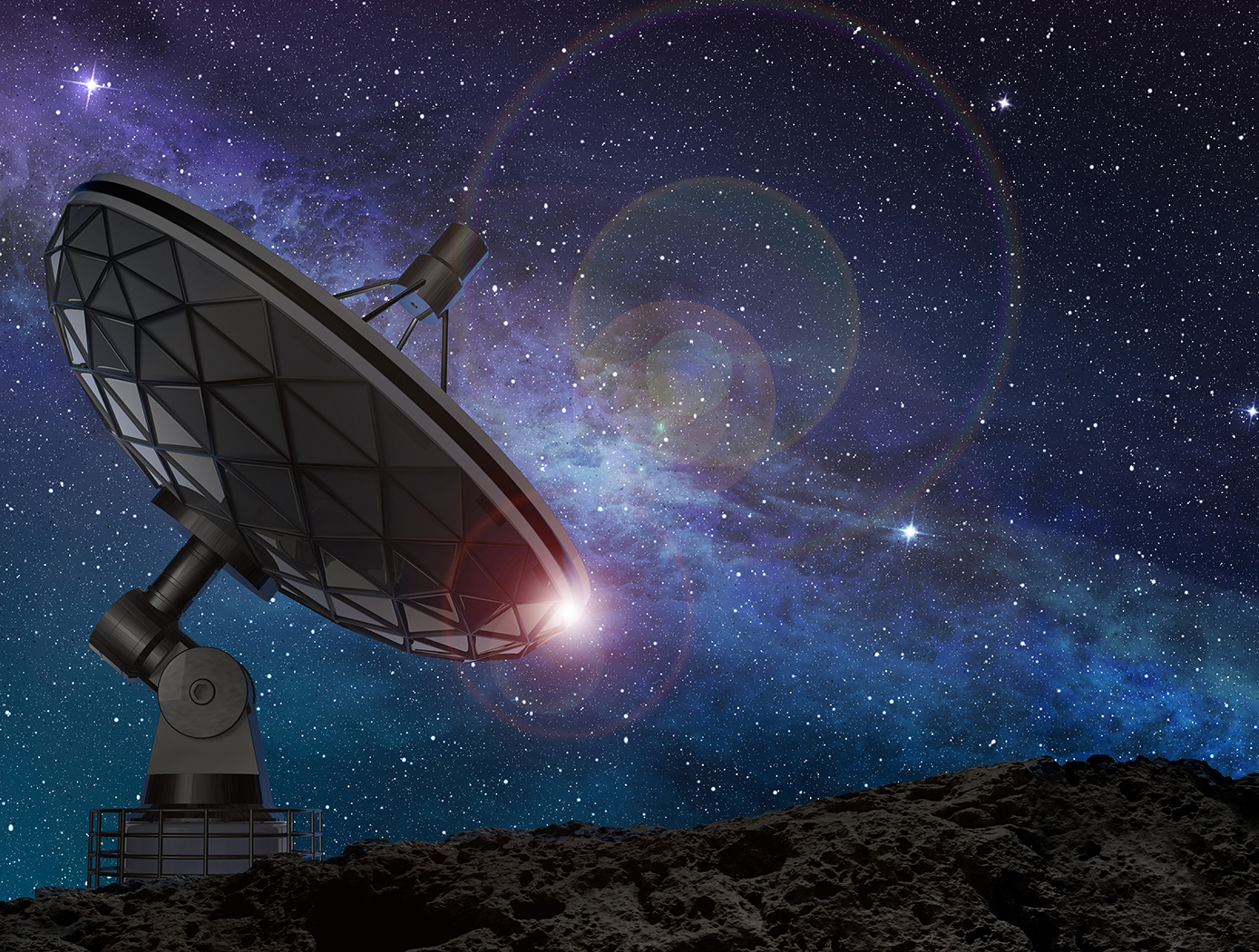
Multimode Directional Coupler
Glenn's researchers originally created the MDC to improve the beacon sources for atmospheric propagation studies. These studies are typically conducted to test atmospheric conditions to determine the signal strength needed for satellite communications. A low-power transmitter (e.g., a beacon source) is attached to the satellite, and transmits a continuous waveform (CW) signal to a receiving station on Earth. However, when a separate frequency is desired, building a new beacon source for the transmitter on the satellite - especially one that will operate at higher frequencies - presents numerous challenges. For one, a single-frequency beacon source requires a temperature-stabilized oscillator for frequency generation separate from that provided by the spacecraft receiver.
To solve such problems, Glenn's innovators fabricated the MDC from two sections of waveguide: a primary waveguide for the fundamental frequency (Ku-band), and a secondary waveguide for the harmonics (Ka-band). These sections are joined together so that precision-machined slots in the second waveguide selectively couple the harmonics, for amplification and transmission. The harmonics can then be used as an additional beacon source with very small power losses to the fundamental signal. Once the separation takes place, the second or higher harmonic can be amplified and transmitted to a station on Earth. The efficiency and performance of the MDC can be optimized through appropriate computer modeling software and currently available high-precision fabrication techniques. Without the complexity and expense involved in building separate traveling wave tube amplifiers to generate additional frequencies, Glenn's MDC enables satellites to produce multiple signals that can be received by multiple stations - a significant leap forward in satellite productivity.
View more patents