Self-Phase-Locked Distributed Gain Laser Architecture
optics
Self-Phase-Locked Distributed Gain Laser Architecture (GSC-TOPS-196)
Provides efficient, passive, and coherent beam combinations from distributed gain sources.
Overview
While both high power and high efficiency laser sources are desired for many applications, limitations on their development and use include power scaling among the many gain materials due to excessive optical intensity. Moreover, as power among many gain elements can be increased, combining the power efficiency has thusfar proven elusive.
The Technology
NASA Goddard Space Flight Center has developed a laser architecture to coherently combine energy from spatially distributed gain sources. Using a combination of lenslet arrays (to split and combine separate beams) or diffractive optical elements, each source can be phase-matched into an effective single source.
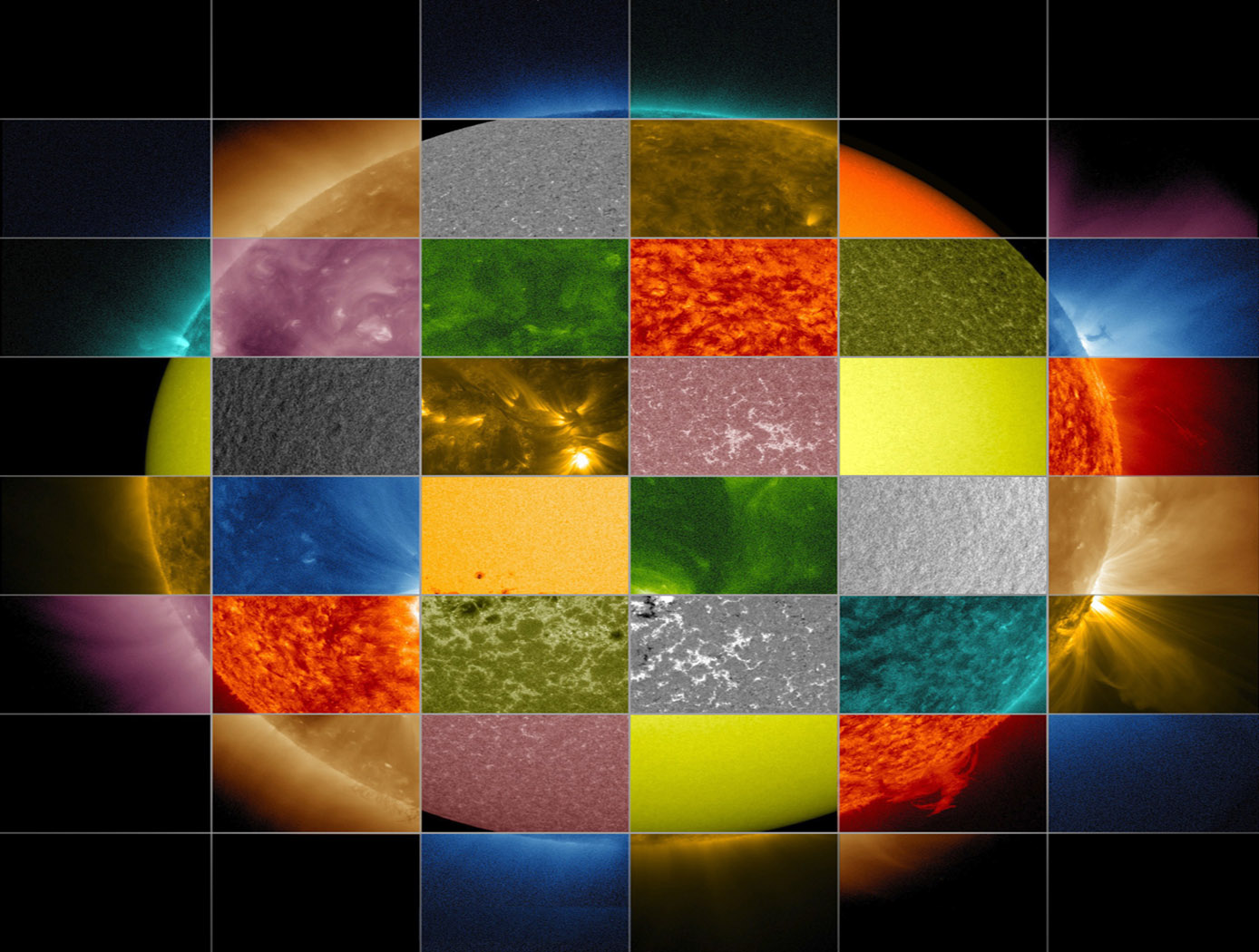
Benefits
- Flexibility in laser system design
- Achieves both higher power and higher efficiency
Applications
- High power laser development
- Semiconductor optics systems
Similar Results

Free-space Fiber Optic Laser Rod
For this new laser concept, a relatively short but large core fiber doped by active lasing material is used in place of a conventional solid state crystal as the amplifier gain media in a free-space configuration. The technology avoids the usual problems of low thresholds for catastrophic optical damage and other nonlinear loss processes in fiber lasers by increasing the fiber core diameter of a standard 9 microns single mode fiber to the order of 1 mm, thereby permitting peak powers to be increased by factors of 10,000. The usual degradation of single mode propagation in large diameter fibers is avoided by keeping fiber lengths short, thereby staying within a free-space single mode propagation regime.

Receiver for Long-distance, Low-backscatter LiDAR
The NASA receiver is specifically designed for use in coherent LiDAR systems that leverage high-energy (i.e., > 1mJ) fiber laser transmitters. Within the receiver, an outgoing laser pulse from the high-energy laser transmitter is precisely manipulated using robust dielectric and coated optics including mirrors, waveplates, a beamsplitter, and a beam expander. These components appropriately condition and direct the high-energy light out of the instrument to the atmosphere for measurement. Lower energy atmospheric backscatter that returns to the system is captured, manipulated, and directed using several of the previously noted high-energy compatible bulk optics. The beam splitter redirects the return signal to mirrors and a waveplate ahead of a mode-matching component that couples the signal to a fiber optic cable that is routed to a 50/50 coupler photodetector. The receiver’s hybrid optic design capitalizes on the advantages of both high-energy bulk optics and fiber optics, resulting in order-of-magnitude enhancement in performance, enhanced functionality, and increased flexibility that make it ideal for long-distance or low-backscatter LiDAR applications.
The related patent is now available to license. Please note that NASA does not manufacturer products itself for commercial sale.

Continuous Wave Laser Source for Injection Seeding
NASA's CW Laser Source for Injection Seeding uses a single laser diode (LD) to produce multiple wavelengths. Depending on the application, the seed laser may or may not be locked to a wavelength reference. For example, in atmospheric differential absorption lidar (DIAL) active remote sensing applications, the seed laser has to be locked and referenced to the species of interest using gas cells. In this context, the seed laser source is first locked to an absorption feature and the generated wavelength is used as a reference from which other offset wavelengths are generated. However, if the requirement calls only to avoid atmospheric absorption then locking may not be required.
Using this new technology, an airborne 2-micron triple pulse integrated path differential absorption (IPDA) LIDAR instrument has been developed at NASA Langley Research Center to measure the column content of atmospheric H2O and CO2 simultaneously and independently. This is achieved by transmitting three successive high-energy pulses, seeded at three different wavelengths, through the atmosphere. The three pulses are emitted 200 microseconds apart and repeated at 50 Hz. The seeding wavelengths were selected to achieve minimum measurement interference from one molecule to the other. Typically, this requires four different CW lasers for seeding. A part of that effort focused on adaptive targeting, which is based on the tuning capability of the on-line wavelength to meet a certain measurement objective depending on observational time and location. The off-line wavelength was assumed constant. The tuning capability can be achieved using the claimed seeding technique using a voltage-controlled oscillator for the on-line and fixed oscillator for the off-line.

Optical Tunable-Based Transmitter for Multiple High-Frequency Bands
NASA Glenn's researchers have developed a means of transporting multiple radio frequency carriers through a common optical beam. In contrast to RF infrastructure systems alone, this type of hybrid RF/optical system can provide a very high data-capacity signal communication and significantly reduce power, volume, and complexity. Based on an optical wavelength division multiplexing (WDM) technique, in which optical wavelengths are generated by a tunable diode laser (TDL), the system enables multiple microwave bands to be combined and transmitted all in one unit. The WDM technique uses a different optical wavelength to carry each separate and independent high-frequency microwave band (e.g., L, C, X, Ku, Ka, Q, or higher bands). Since each RF carrier operates at a different optical wavelength, the tunable diode laser can, with the use of an electronic tunable laser controller unit, adjust the spacing wavelength and thereby minimize any crosstalk effect.
Glenn's novel design features a tunable laser, configured to generate multiple optical wavelengths, along with an optical transmitter. The optical transmitter modulates each of the optical wavelengths with a corresponding RF band and then encodes each of the modulated optical wavelengths onto a single laser beam. In this way, the system can transmit multiple radio frequency bands using a single laser beam. Glenn's groundbreaking concept can greatly improve the system flexibility and scalability - not to mention the cost of - both ground and space communications.

Thermally-Adaptive Solid State Laser Crystal Mount
NASA’s laser mount technology introduces a unique flexible crystal mount to accommodate the dynamics of thermal expansion to eliminate unsymmetrical thermally induced mechanical stresses on the crystal. In addition, while the mount accommodates thermal expansion, it also offers fixed placement of the crystal to maintain alignment and provides continuous and uniform surface contact between the mount and crystal for rapid dissipation of heat. The mount is compatible with any heat sink reservoir.
The mount design allows unrestrained thermal expansion of the crystal in two dimensions (i.e. a- and c- axes) because of the design shown in the figure below.
The L-shape blocks also deliver cooling to the crystal by providing a path to the heat sink reservoir. The L-shape blocks are manufactured with a high thermal conductivity material such as copper. A softer material with high thermal conductivity such as indium is used to buffer the interface between the crystal and the L-shape blocks surfaces. A coolant medium acts to transfer the heat from the crystal to the cooled mount. Cooling can be provided in different ways – for example by water or by heat pipes with radiator (for use in space). The springs used to hold the laser crystal also provide the adjustment method to align the beam, and once aligned, the crystal mount is very stable.
The related patent is now available to license. Please note that NASA does not manufacture products itself for commercial sale.