Cavity Enhanced Absorption Spectroscopy (CEAS) for Ozone Detection
Sensors
Cavity Enhanced Absorption Spectroscopy (CEAS) for Ozone Detection (GSC-TOPS-214)
A pulsed light emitting diode (LED) configured to emit a light towards a photomultiplier tube (PMT).
Overview
Engineers at NASA's Goddard Space Flight Center tasked with measuring atmospheric Ozone, an important pollutant and greenhouse gas, quickly discovered that existing optical instrumentation would not be sufficient to achieve their performance requirements. Specifically, the current systems did not possess the precision necessary to make low concentration measurements at fast time resolution. This innovation utilizes Cavity Enhanced Absorption Spectroscopy (CEAS) with a simple and inexpensive optical and electrical design to overcome these limitations and achieve high quality Ozone measurements. The sensitivity of the instrument developed using CEAS exceeds that of commercially available instrumentations, and matches performance of complex chemiluminescence instruments.
The Technology
This technique takes advantage of Ozone's strong absorption at specific wavelengths in the UltraViolet (UV). To take measurements, UV light is projected through a cell bounded by highly reflective mirrors. These mirrors increase the path length of the cell by inducing the light to bounce back and forth within the cell, increasing the likelihood of Ozone absorption within the cell. Finally, an absorption measurement is taken via a detector outside the cell and opposite of the UV source. This innovation produces an ozone detection precision of 0.12 parts per billion (ppb) in 0.1 second integration. This corresponds to 0.012 ppb in ten seconds integration.
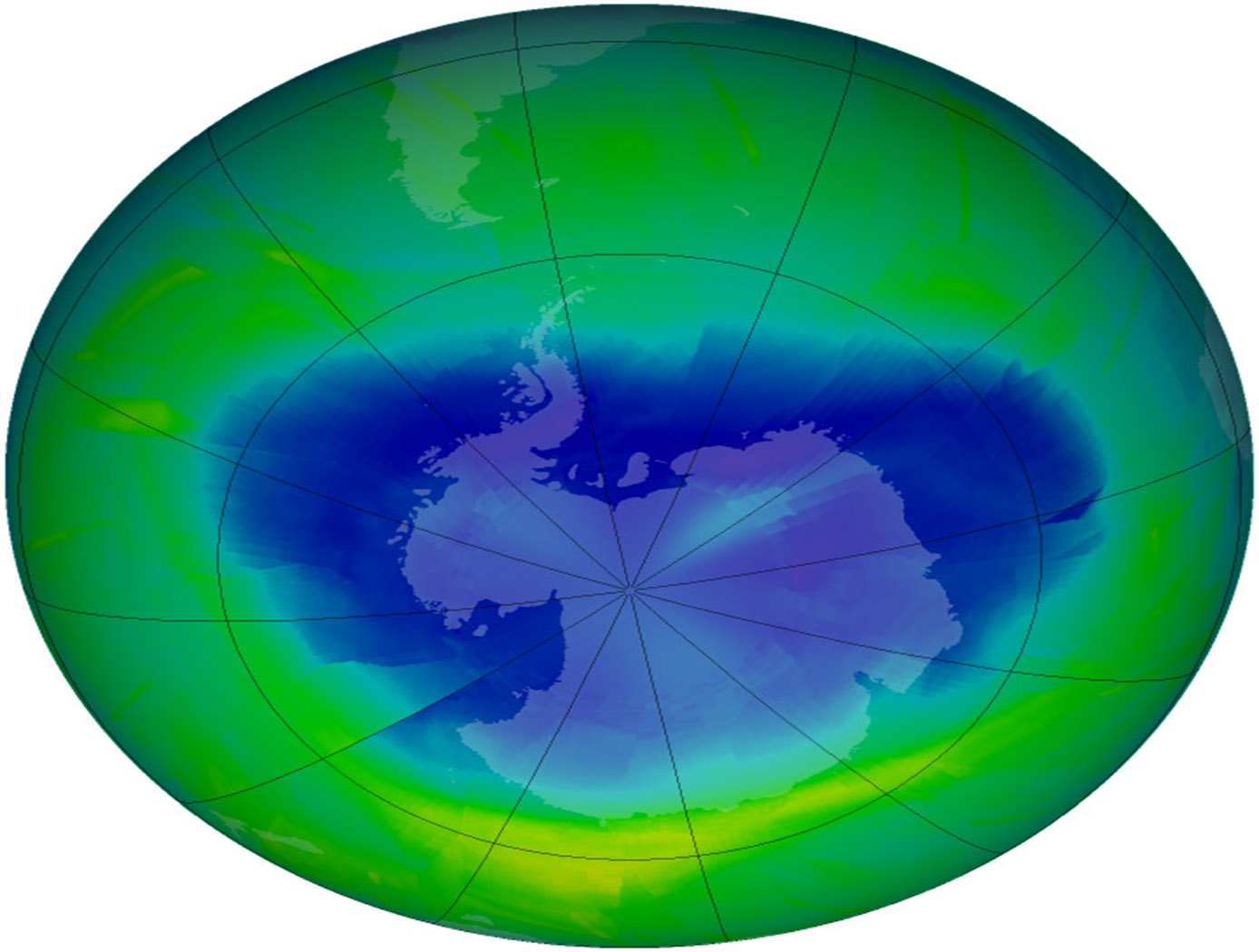
Benefits
- High precision allows detection of low concentrations
- Fast time resolutions
- Reduced parts lead to simple and inexpensive
Applications
- Spectroscopy
- Environmental Monitoring
Similar Results

Wideband, Subharmonic Mixer for Spectrometers
The novel mixer offers wideband and sub-harmonic conversion capabilities for enhanced signal processing across a broad frequency range. The mixer operates at 470-600 GHz and includes a LO waveguide to allow 265-300 GHz input signal and a radio frequency (RF) waveguide for the 470-600 GHz operation. The LO and RF signal multiply and down-convert the RF signal to an IF signal to a much lower frequencies for further digitization. The mixer is designed on a gold and quartz substrate for a lower dielectric constant. The filter design uses a triangular patch resonator-based low-pass filter to reduce the size of the mixer as well as isolates the LO signal and the wide IF signal. Additionally, an IF filter, RF filter, Schottky diode, LO, and RF probes are integrated into a single chip to further reduce the dimensions of the mixer. The invention also leverages an antiparallel diode orientation, where the LO frequency is half of the RF input. This LO signal is amplified and multiplied up to 265-300 GHz to provide an input power of 3-5 mW to pump the antiparallel mixer.
The technology offers significant advantages in remote sensing and high-speed communications, enabling simultaneous detection of multiple molecular species and enhancing the efficiency of submillimeter-wave heterodyne spectrometers. The wideband functionality achieves high data rates required in emerging 6G networks and offers exceptional sensitivity, with prototype tests showing a conversion loss below 12 dB and noise temperatures under 4000 K at 470 GHz. The integration of components such as filters and diodes into a single chip reduces system size and complexity, contrasting with traditional multi-chip setups. The design is scalable across frequencies from 1 GHz-1 THz with minimal modifications, with the system's form factor inversely scaling with frequency. These features make the technology versatile for applications in environmental monitoring, planetary exploration, radar systems, and advanced communication systems.

Photo-Acoustic Sub Part-Per-Billion Chemical Sensing
The technology is a sensor for remotely detecting sub part-per-billion (ppb) levels of ambient trace gases and chemical species. The system includes a high-repetition-rate, pulsed laser module that is spectrally tuned to a desired chemical species. The photons from the laser are absorbed by the target chemical, creating an acoustic vibration that impacts a diaphragm (which acts like a speaker). A highly sensitive, photo-emf detector is then used to measure the magnitude of the vibration, which corresponds to the concentration of the target chemical. The technology is being developed for NASA's trace-gas measurement needs for validation and ground truth studies to support airborne and space-based LIDAR operations. The technology has application as a chemical sniffer to detect hazardous or toxic chemical species in the vicinity of IEDs, explosives, or other chemical agents. In such an application the sensor could detect chemical species hidden inside closed containers, bags, or car trunks.

Miniaturized Laser Heterodyne Radiometer
This instrument uses a variation of laser heterodyne radiometer (LHR) to measure the concentration of trace gases in the atmosphere by measuring their absorption of sunlight in the infrared. Each absorption signal is mixed with laser light (the local oscillator) at a near-by frequency in a fast photoreceiver. The resulting beat signal is sensitive to changes in absorption, and located at an easier-to-process RF frequency. By separating the signal into a RF filter bank, trace gas concentrations can be found as a function of altitude.

Multi-Spectral Imaging Pyrometer
This NASA technology transforms a conventional infrared (IR) imaging system into a multi-wavelength imaging pyrometer using a tunable optical filter. The actively tunable optical filter is based on an exotic phase-change material (PCM) which exhibits a large reversible refractive index shift through an applied energetic stimulus. This change is non-volatile, and no additional energy is required to maintain its state once set. The filter is placed between the scene and the imaging sensor and switched between user selected center-wavelengths to create a series of single-wavelength, monochromatic, two-dimensional images. At the pixel level, the intensity values of these monochromatic images represent the wavelength-dependent, blackbody energy emitted by the object due to its temperature. Ratioing the measured spectral irradiance for each wavelength yields emissivity-independent temperature data at each pixel. The filter’s Center Wavelength (CWL) and Full Width Half Maximum (FWHM), which are related to the quality factor (Q) of the filter, are actively tunable on the order of nanoseconds-microseconds (GHz-MHz). This behavior is electronically controlled and can be operated time-sequentially (on a nanosecond time scale) in the control electronics, a capability not possible with conventional optical filtering technologies.

Fast and widely tunable monolithic optical parametric oscillator for laser spectrometer
NASA Goddard Space Flight Center has developed a faster and widely-tunable monolithic optical parametric oscillator for use in laser spectrometers. This technology provides a continuously-tunable spectrum across any target, adding flexibility to the overall instrument. In addition, only 1 nonlinear crystal and oscillator pump source are used, greatly simplifying the spectrometer system.