Macroflash (Cup Cryostat)
Instrumentation
Macroflash (Cup Cryostat) (KSC-TOPS-22)
A compact instrument to measure thermal conductivity of materials at below-ambient temperatures
Overview
NASA Kennedy Space Center seeks partners interested in a practical method for measuring thermal performance of materials at below-ambient temperatures or subjected to a large temperature difference. The technology uses liquid nitrogen as a direct heat energy meter and is applicable to testing at a wide range of temperatures from 373 K down to 77 K and under an ambient pressure environment.
The Macroflash follows the guidelines of the newly established technical standard ASTM C1774 (Annex A4) and provides a cost-effective, field-representative methodology to test any material for below-ambient temperature applications. From engineered systems to research testing to quality control in manufacturing, the technology provides utility for the fields of energy, transportation, construction, and environment.
The Technology
Advances in new polymers and composites along with growing industrial needs in below-ambient temperature applications have brought about the Macroflash development. Accurate thermal performance information, including effective thermal conductivity data, are needed under relevant end-use conditions. The Macroflash is a practical tool for basic testing of common materials or research evaluation of advanced materials/systems.
The Macroflash can test solids, foams, or powders that are homogeneous or layered in composition. Test specimens are typically 75mm in diameter and 6mm in thickness. The cold side is maintained by liquid nitrogen at 77 K while a heater disk maintains a steady warm-side temperature from ambient up to 373 K. The steady boiloff of the liquid nitrogen provides a direct measure of the heat energy transferred through the thickness of the test specimen. Nitrogen or other gas is supplied to the instrument to establish a stable, moisture-free, ambient pressure environment. Different compression loading levels can also be conveniently applied to the test specimen as needed for accurate, field-representative thermal performance data. The Macroflash is calibrated from approximately 10 mW/m-K to 800 mW/m-K using well-characterized materials.
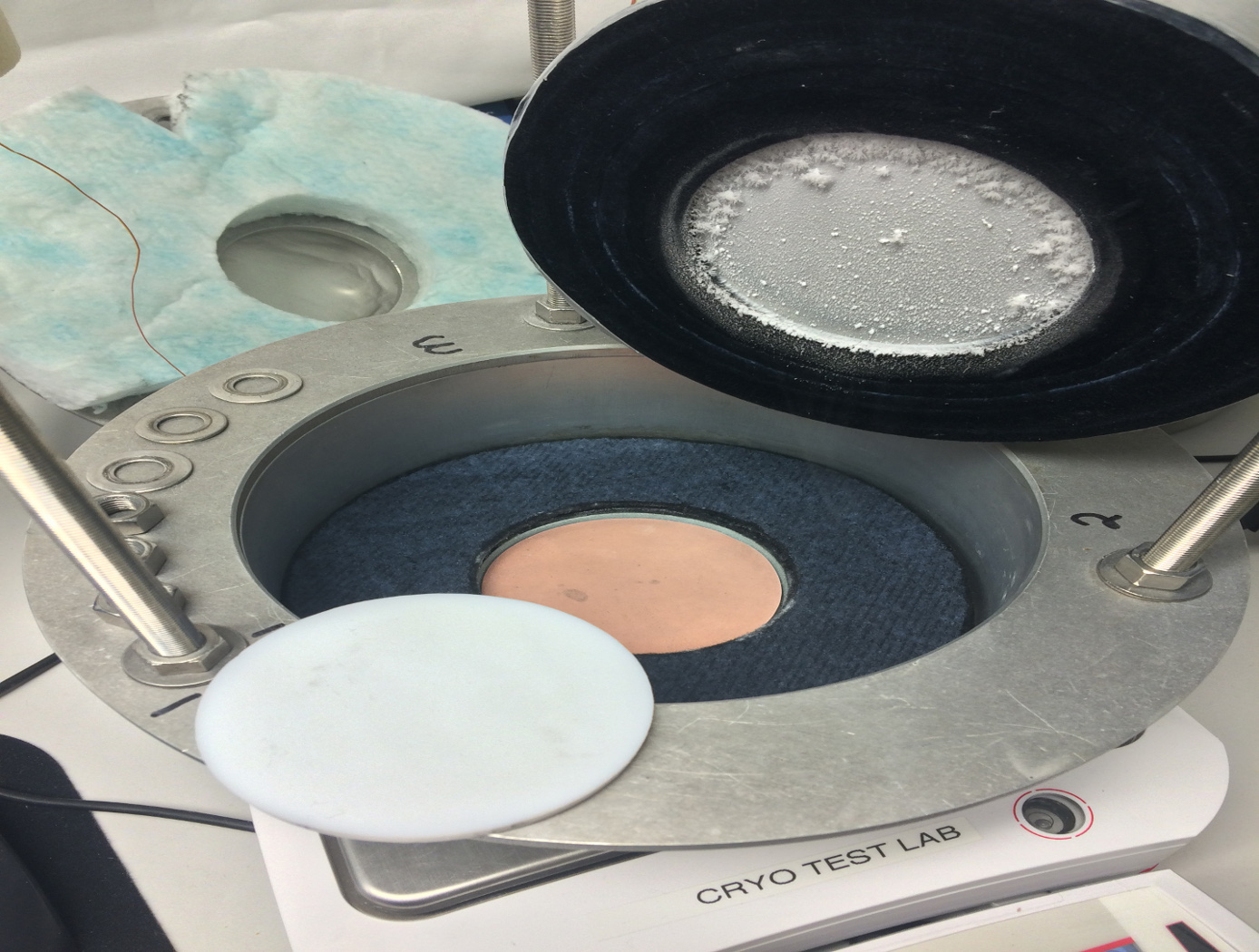
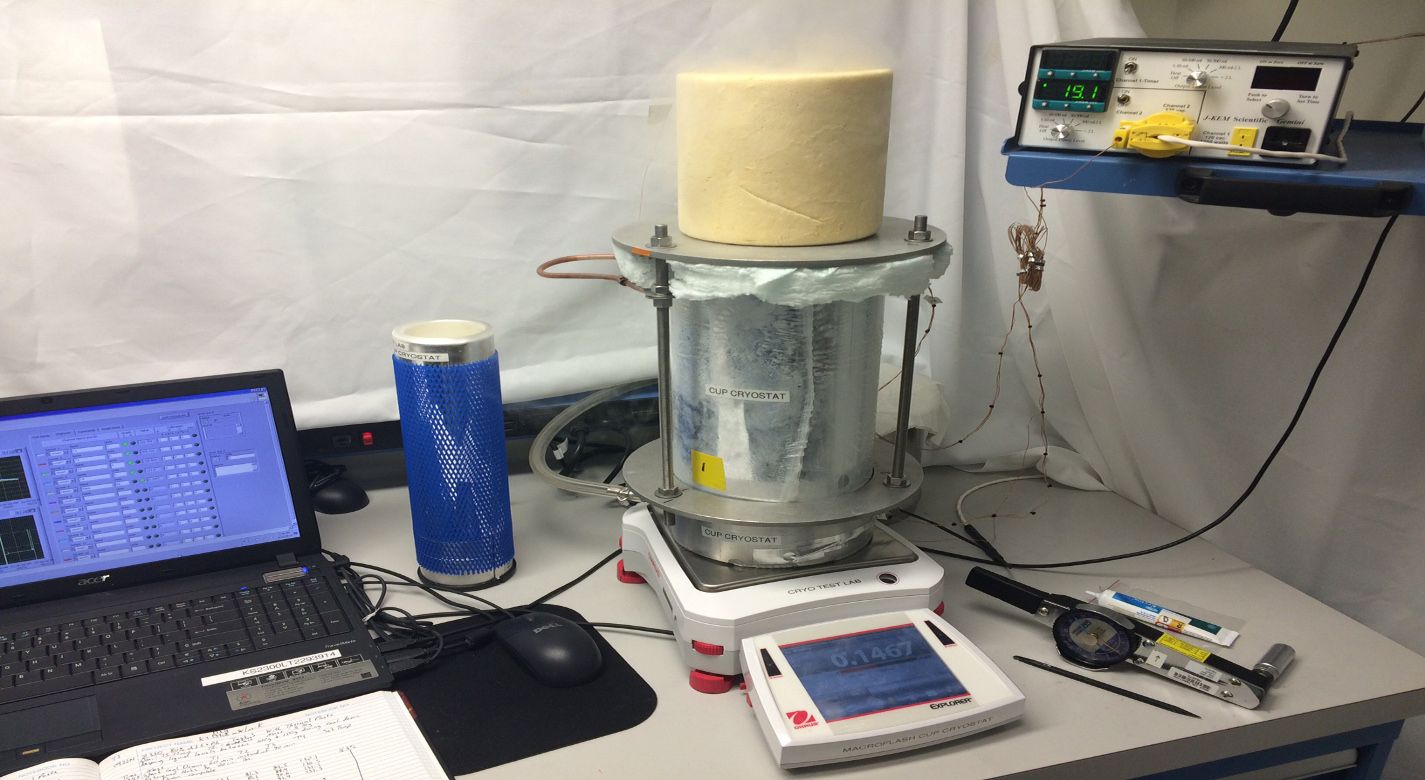
Benefits
- Compact and easy to use
- Cost-effective compared to commercially available thermal test instruments
- Convenient size for test specimens (solids, foams, or powders)
- Multiple tests can be completed in one day
- No costly vacuum chamber set-up required
- Provides thermal conductivity data for below-ambient temperatures and under more realistic conditions of a large temperature difference
Applications
- Electrical power and energy storage
- Refrigeration and cryogens
- Aerospace and advanced materials
- Construction materials
- Ground and air transportation
Similar Results

Cryostat-100
Cryostat-100 combines the best features of previous cryostats developed by NASA, while offering new features and conveniences. This unit can readily handle the full range of cryogenic-vacuum conditions over several orders of magnitude of heat flux. Guide rings, handling tools, and other design items make insulation change-out and test measurement verification highly reliable and efficient to operate. The new apparatus requires less ancillary equipment (it is not connected to storage tank, phase separator, subcooler, etc.) to operate properly. It is top-loading, which makes disassembly, change-out, and instrumentation hook-up much faster. The thermal stability is improved because of internal vapor plates, a single-tube system of filling and venting, bellows feed-throughs, Kevlar thread suspensions, and heavy-wall stainless-steel construction.
The cold mass of Cryostat-100 is 1m long, with a diameter of 168 mm. The test articles can therefore be of a corresponding length and diameter, with a nominal thickness of 25.4 mm. Shorter lengths are acceptable, and thicknesses may be from 0 mm to 50 mm. Tests are conducted from ambient pressure (760 torr) to high vacuum (below 110-4 torr) and at any vacuum pressure increment between these two extremes. The residual gas (and purge gas) is typically nitrogen but can be any purge gas, such as helium, argon, or carbon dioxide.
Typically, eight cold vacuum pressures are performed for each test series. The warm boundary temperature is approximately 293 K, and the cold boundary temperature is approximately 78 K. The delta temperature for the cryogenic testing is therefore approximately 215 K. A unique lift mechanism provides for change-out of the insulation test specimens. It also provides for maintenance and other operations in the most effective and time-efficient ways. The lift mechanism is also a key to the modularity of the overall system.

Cryostat-500
The Cryostat-500 provides laboratory measurement of the steady-state thermal transmission properties of thermal insulation systems under conditions below ambient temperature. Liquid nitrogen is used as a direct measure of the energy going through the test specimen. Thermal insulation systems may be composed of one or more materials that may be homogeneous or non-homogeneous at boundary conditions from 77 K to 373 K and in environments from high vacuum (10E-7 torr) to ambient pressure (10E+3 torr).
The Cryostat-500 provides a much wider range of thermal performance and covers the full range of environmental conditions for applications below ambient temperature. The instrument has been proven through extensive testing of foams, composite panels, multilayer insulation (MLI) systems, aerogel blankets, fiberglass, and many other types of materials. Both the quality and quantity of the thermal performance data for insulation materials and systems have increased even as the process and method has become more time efficient and cost effective. Further guidelines on the test method and equipment for the Cryostat-500 are given in ASTM C1774, Annex A3.
Feedthrough for Severe Environments and Temperatures
Space and ground launch support related hardware often operate under extreme pressure, temperature, and corrosive conditions. When dealing with this type of equipment, it is frequently necessary to run wiring, tubes, or fibers through a barrier separating one process from another with one or both operating in extreme environments. Feedthroughs used to route the wiring, tubes, or fibers through these barriers must meet stringent sealing and leak tightness requirements.
This affordable NASA feedthrough meets or exceeds all sealing and leak requirements utilizing easy-to-assemble commercial-off-the-shelf hardware with no special tooling. The feedthrough is a fully reconfigurable design; however, it can also be produced as a permanent device. Thermal cycling and helium mass spectrometer leak testing under extreme conditions of full cryogenic temperatures and high vacuum have proven the sealing capability of this feedthrough with or without potting (epoxy fill) on the ends. Packing material disks used in the construction of the device can be replaced as needed for rebuilding a given feedthrough for another job or a different set of feeds if potting is not used for the original feedthrough build. (Potting on one or both sides of the sleeve provides double or triple leak sealing protection). Variable Compression Ratio (VCR) connectors were adapted for the pressure seal on the feedthrough; however, any commercial connector can be similarly adapted. The design can easily be scaled up to larger (2" diameter) and even very large (12" or more) sizes.

Thin Film Sensor for Ultra High-Temp Measurement
The thin film sensor’s principal advantage lies in its potential to take high frequency temperature measurements from the surface of a reentering spacecraft while simultaneously withstanding the high temperature and oxidizing environment encountered. This data provides engineers with operational phase measurements used to refine the spacecraft’s operational envelope and track flight hardware behavior in addition to providing high frequency temperature measurements that can inform the physics of a boundary layer.
Mismatches in coefficients of thermal expansion (CTE) are expected in TPS-based sensor applications because the metallic materials used for temperature sensing have thermal expansion rates that differ from the rates of the substrate and coating materials in the TPS. At high temperatures during reentry, this mismatch in CTE can create a significant strain differential between the metallic sensor, sensor leads, and the materials to which the sensor and leads are bonded.
High frequency response temperature measurements on the surface of entry spacecraft are not currently possible above ~700 F with existing measurement capabilities. This shortcoming is primarily due to the need for robust sensor behavior at temperatures of several thousand degrees F. The sensor design of this technology preserves the integrity of sensor components while enhancing its high temperature functionality.
The thin film temperature sensor has a technology readiness level (TRL) 5 (Component and/or breadboard validation in relevant environment) and is now available for patent licensing. Please note that NASA does not manufacture products itself for commercial sale.

Adaptive Thermal Management System
Efficient thermal management has long been an issue in both commercial systems and in the extreme environments of space. In space exploration and habitation, significant challenges are experienced in providing fluid support systems such as cryogenic storage, life support, and habitats; or thermal control systems for launch vehicle protection, environmental heat management, or electronic instruments. Furthermore, these systems operate in dynamic, transient modes and often under extremes of temperature or pressure. The current technical requirements associated with the thermal management of these systems result in control issues as well as significant life-cycle costs.
To combat these issues, the Adaptive Thermal Management System (ATMS) was developed to help provide the capability for tanks, structural walls, or composite substrate materials to switch functionality (conductive or insulative) depending on environmental conditions or applied stimuli. As a result, the ATMS provides the ability to adapt between both heating and cooling modes within a single system. For example, shape memory alloy (SMA) elements are used to actuate at certain design temperatures to create a conductive bridge between two metal plates allowing broad-area heat rejection from the hotter surface. Upon cooling to the lower design set-point, the SMA elements return to their original shapes, thereby breaking the conductive path and returning the system to its overall insulative state.
This technology has the potential to be applied to any system that would have the need for a self-regulating thermal management system that allows for heat transfer from one side to another.