Electron Beam Free Form Fabrication (EBF3)
Manufacturing
Electron Beam Free Form Fabrication (EBF3) (LAR-TOPS-164)
Metal deposition apparatus to build metallic components directly from CAD
Overview
Electron Beam Freeform Fabrication, or EBF3, is a process that uses an electron beam gun, a dual wire feed and computer controls to manufacture metallic structures for building parts or tools in hours, rather than days or weeks. EBF3 can manufacture complex geometries in a single operation and provides efficient use of power and feedstock. The technology has a wide range of applications, including automotive, aerospace, and rapid prototyping. It can build large metallic parts measuring feet in length, and has been reduced in size and power to enable 0-gravity experiments conducted on NASA's Reduced Gravity aircraft.
The Technology
EBF3 works in a vacuum chamber, where an electron beam is focused on a constantly feeding source of metal wire, which is melted and applied by drawing one layer at a time on a computer-controlled positioning table until the part is complete. By controlling the raster pattern, the e-beam selectively heats the outer edges of the wire if it strays from the melt zone. With such selective heating, the wire automatically curls away from the high heat outer edge and back into the region of the molten pool. Thus, with a fixed raster pattern, the process becomes self-correcting without any sensing or external control of process parameters. EBF3 can handle two different sources of metal feedstock at the same time, which can be controlled independently to allow precise control of mixing of materials during deposition.
EBF3 incorporates the following technologies, which can also be licensed individually: (1) a vapor-barrier vacuum isolation system that maintains a vacuum in the electron beam column adjoined to another chamber at higher pressure without loss of vacuum; (2) a system that controls and measures the height of an additive layer of metal using an electron beam in combination with a sensor and image processor; (3) a gas phase alloying system for wire fed joining & deposition, which is being developed for the controlled introduction of gases into molten metals in order to selectively impart beneficial properties to the final product (e.g. strength, corrosion resistance, etc.); and (4) a closed-loop system that is being developed to detect various fabrication anomalies and automatically send feedback to the fabrication system to modify the input parameters to maintain process consistency.
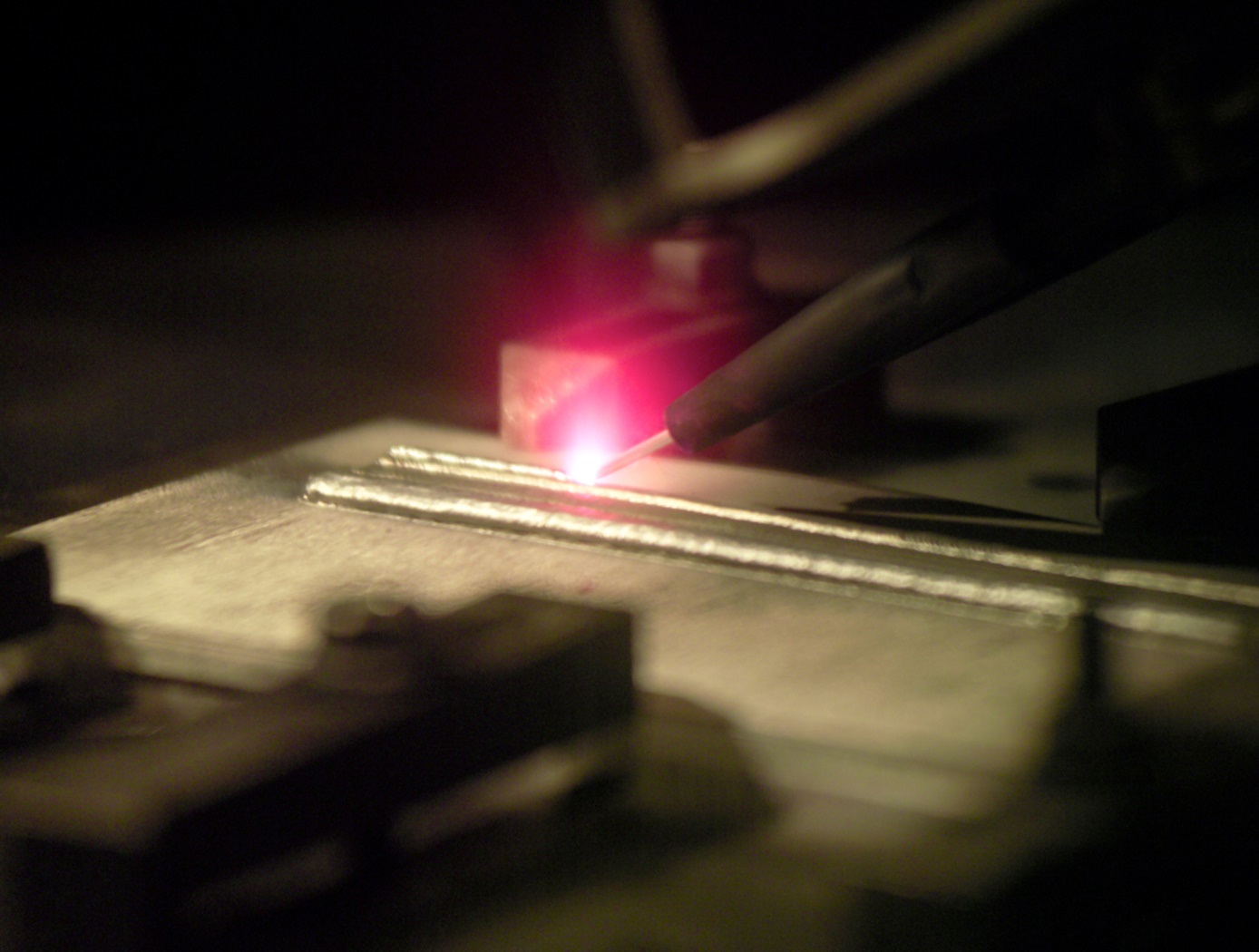
Benefits
- Self-correcting stabilization and optimization of e-beam during the deposition process
- Provides continuous and predictable deposition pattern
- Simplifies deposition of complex geometries
- Optimizes microstructural control of the solidified molten metal
- Provides efficient use of power and feedstock
- Improves automated operation
Applications
- Welding of metal structures for automotive, aerospace, and other industrial and commercial manufacturing
- Free-form fabrication of complex metal components in remote locations
- Near-net shape manufacturing and rapid prototyping
- Applicable to variety of metal fabrication markets, from automotive and aerospace to sporting goods and medical devices
Technology Details
Manufacturing
LAR-TOPS-164
LAR-18183-1
LAR-18871-1
LAR-18871-1
Similar Results

Solid State Sensor for Detection and Characterization of Electric Fields
This equilibrium-reversing-gate field effect transistor (ergFET) deploys an electrode near the gate of the transistor to control and reverse leakage currents which are typical in transistors and can lead to measurement errors. It can be built into an array to enable higher resolution imaging and is a solid state device free of moving parts. This enables portable and hand held sensor designs.

Novel Overhang Support Designs for Powder-Based Electron Beam Additive Manufacturing (EBAM)
EBAM technology is capable of making full-density, functional metallic components for numerous engineering applications; the technology is particularly advantageous in the aerospace, automotive, and biomedical industries where high-value, low-volume, custom-design productions are required. A key challenge in EBAM is overcoming deformation of overhangs that are the result of severe thermal gradients generated by the poor thermal conductivity of metallic powders used in the fabrication process. Conventional support structures (Figure 1a) address the deformation challenge; however, they are bonded to the component and need to be removed in post- processing using a mechanical tool. This process is laborious, time consuming, and degrades the surface quality of the product.
The invented support design (Figure 1b) fabricates a support underneath an overhang by building the support up from the build plate and placing a support surface underneath an overhang with a certain gap (no contact with overhang). The technology deposits one or more layers of un-melted metallic powder in an elongate gap between an upper horizontal surface of the support structure and a lower surface of the overhang geometry. The support structure acts as a heat sink to enhance heat transfer and reduce the temperature and thermal gradients. Because the support structure is not connected to the part, the support structure can be removed freely without any post-processing step.
Future work will compare experimental data with simulation results in order to validate process models as well as to study process parameter effects on the thermal characteristics of the EBAM process.

Electroactive Scaffold
Current scaffold designs and materials do not provide all of the appropriate cues necessary to mimic in-vivo conditions for tissue engineering and stem cell engineering applications. It has been hypothesized that many biomaterials, such as bone, muscle, brain and heart tissue exhibit piezoelectric and ferroelectric properties. Typical cell seeding environments incorporate biochemical cues and more recently mechanical stimuli, however, electrical cues have just recently been incorporated in standard in-vitro examinations. In order to develop their potential further, novel scaffolds are required to provide adequate cues in the in-vitro environment to direct stem cells to differentiate down controlled pathways or develop novel tissue constructs. This invention is for a scaffold that provides for such cues by mimicking the native biological environment, including biochemical, topographical, mechanical and electrical cues.

Thin-Films with Integrated Structural and Functional Elements
The technology uses additive print manufacturing to produce hierarchical and integrated structural and functional elements into large-area thin-film structures. Adding these structural and functional elements has the potential to enable very lightweight, large-scale thin-films with improved damage tolerance, self-deployment capability, flexibility, and multifunctional (optical, thermal, electrical) connectivity and interrogation capabilities. Based on simple and proven additive manufacturing concepts, advanced geometrical, biomimetic (insect wing), and hierarchical structures could be applied to, or eventually with further development integrated within the bulk of large-area thin films using roll-to-roll processing techniques for a potentially low-cost manufacturing approach. The subject technology potentially addresses many of the disadvantages of current large-scale membrane material systems, which are prone to damage or require extensive deployment and support structures.

Automated Fabric Circuit and Antenna Fabrication
Modern production of e-textiles utilizes an embroidery technique called “e-broidery” that directly stitches circuit patterns with conductive thread onto textiles. This automated manufacturing process combines steps of e-broidery and milling to expand the application of e-textiles to high-current and high-speed uses. Manufacturing begins with two layouts of the desired conductive pattern. After assembling the layers of conductive and nonconductive materials, e-broidery is performed with the second layout and nonconductive thread to secure the layers together and designate the pattern for the conductive material. The secured assembly is transferred to an automated milling or laser cutting machine, which cuts the desired conductive pattern and releases the unneeded portions of the conductive material. The resulting e-textiles are tightly woven together, providing higher surface conductivity and impedance control. Initial comparison tests assessing the performance of fabric-based spiral antennas developed with this method, compared to conventional antennas, indicated no loss in performance across multiple metrics, including voltage standing wave ratio (VSWR), radiation pattern, and axial ratio performance.
The Method and Apparatus for Fabric Circuits and Antennas is a technology readiness level (TRL) 6 (system/subsystem prototype demonstration in a relevant environment). The innovation is now available for your company to license. Please note that NASA does not manufacture products itself for commercial sale.