Premixed, High-Pressure, Multi-Fuel Burner
power generation and storage
Premixed, High-Pressure, Multi-Fuel Burner (LEW-TOPS-134)
Operates on a variety of gaseous fuels and oxidizers
Overview
NASA's Glenn Research Center has developed a novel design for a fully premixed high-pressure burner capable of operating on a variety of gaseous fuels and oxidizers, including hydrogen-air mixtures, with a low pressure drop. The burner provides a rapidly and uniformly mixed fuel-oxidizer mixture that is suitable for use in a fully-premixed combustion regime that has the benefit of low pollutant emissions. Further, it is free from harmful flashback effects, combustion instabilities, and thermal meltdown problems that are normally associated with premixed combustion systems operating at high pressures. This burner can be easily scaled for use in practical low-emissions combustion systems such as stationary power plants or hydrogen-air combustion for vehicles. This technology is also applicable to process gas heaters, chemical processing, process gas afterburners, kiln or furnace burners, utility boiler burners, gas reforming burners, and fuel cell processing burners.
The Technology
NASA Glenn's fully premixed burner design accomplishes the rapid mixing of the fuel and air flows while simultaneously providing backside impingement cooling to the burner face. This novel burner technology has been demonstrated to operate on hydrogen-air mixtures at pressures up to 30 bar, and at equivalence ratios (Phi) ranging from 0.15 to 5.0, but typically at equivalence ratios below 0.6 or above 2.0 for extended periods of time. It has also been demonstrated to work well with hydrogen-carbon monoxide fuel mixtures in a 1:1 mixture (by volume). The design provides a uniform zone of combustion products and temperatures, and is able to achieve complete and rapid mixing of the reactant gases over a distance as short as 5 mm, with the combustion products attaining a fully-reacted state within about 10 mm downstream of the burner face. Effectiveness of the mixing is not dependent on the use of hydrogen gas, therefore the system works well for other gaseous fuels such as methane, propane, or natural gas, in a fully premixed mode.
The design of the Glenn's burner is simple and straightforward to manufacture using conventional techniques. The modular design of the burner lends itself to scalability for larger power output applications. This burner is simple to operate and is robust for use in an industrial setting such as low-emissions stationary gas turbine engine, or for aircraft gas turbine engines.
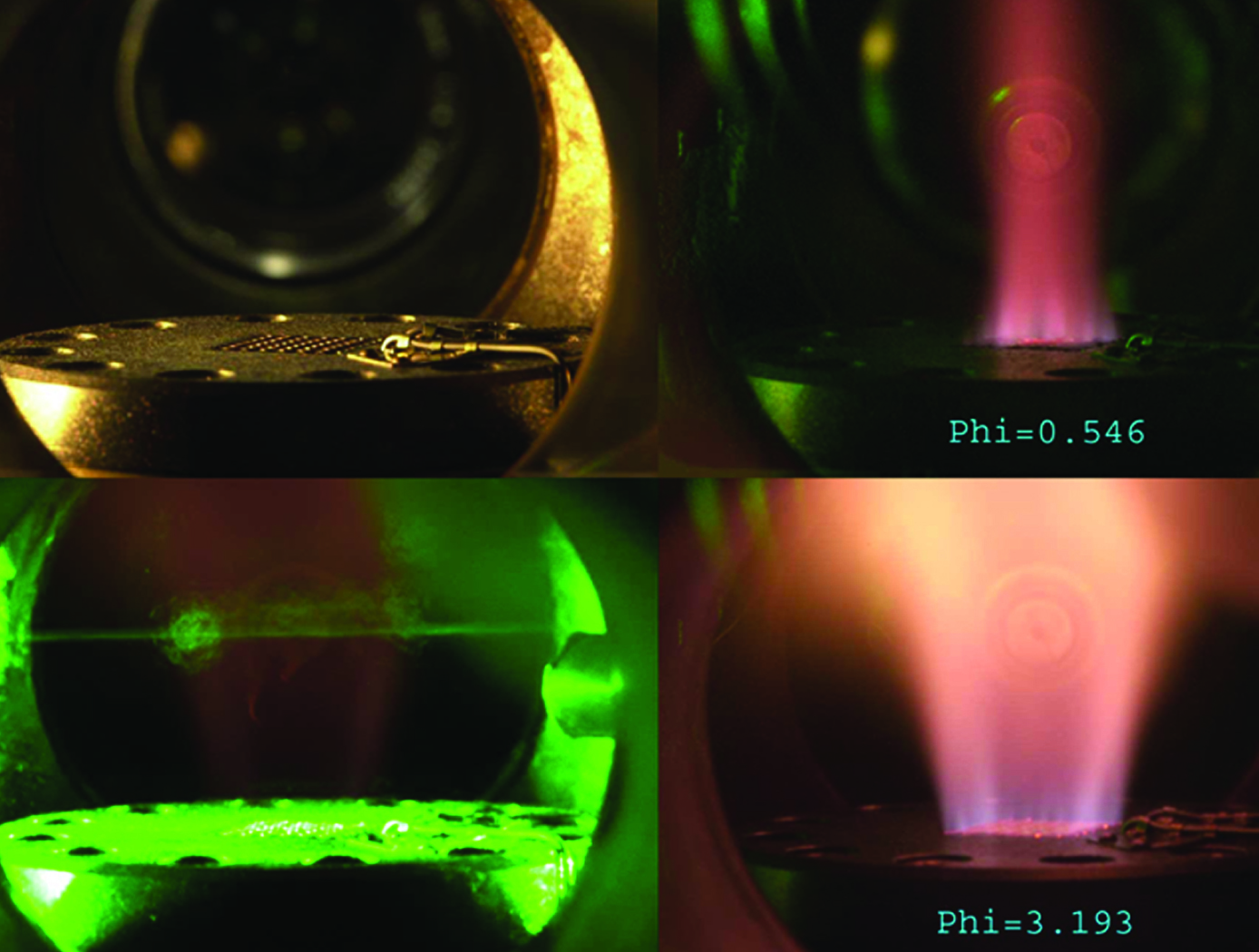
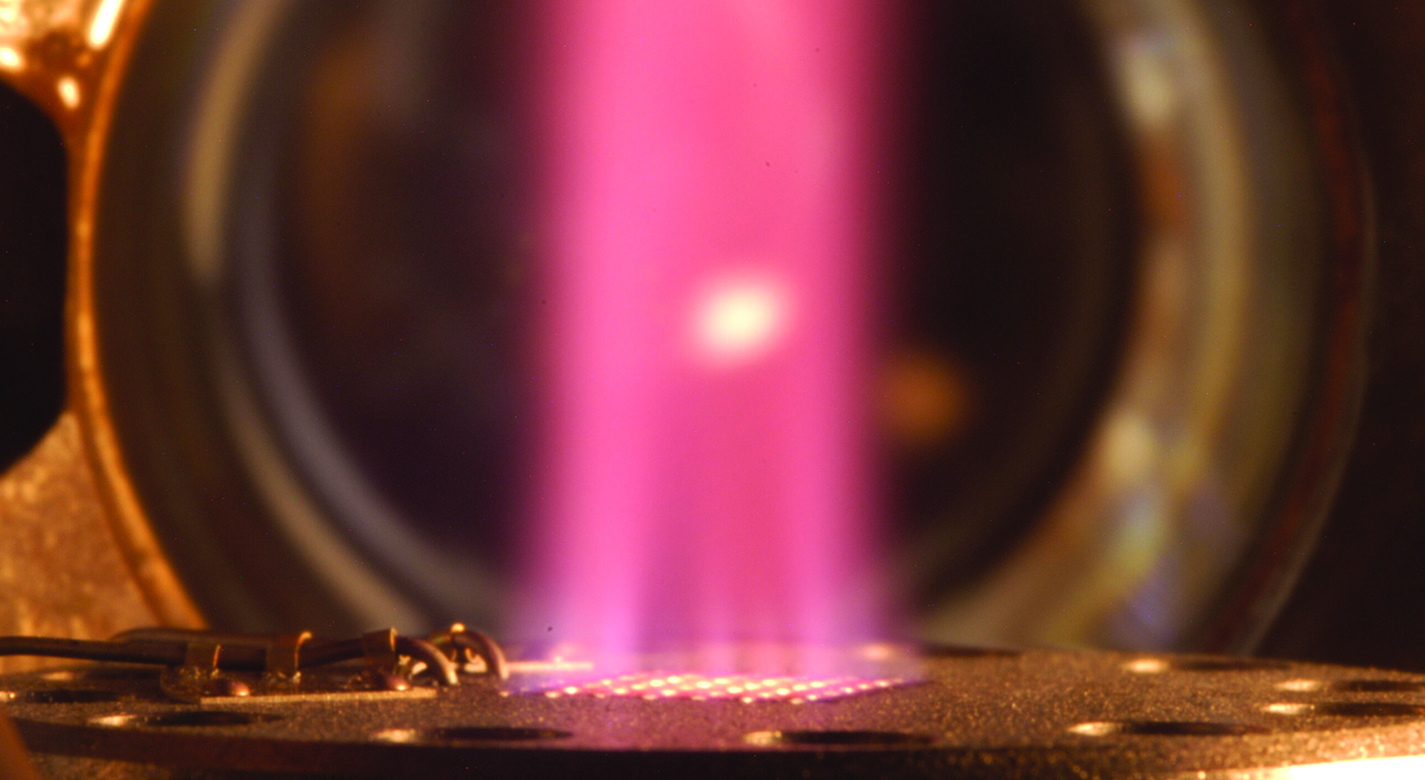
Benefits
- Robust: Operates on a variety of gaseous fuels and oxidizers
- Efficient: Provides a uniform zone of combustion products and temperatures and achieves complete and rapid mixing
- Stable: Expansion ratio helps to stabilize flame and provide more uniform flame zone
- Longer life: Self-cooled design
- Scalable: Offers a modular design that lends itself to scalability for larger power output applications
- Easy to manufacture: Features a design that is straightforward to manufacture with conventional techniques or with advanced methods
- Environmentally friendly: Lower fuel consumption and reduced emissions
- Safer: Operates at high pressures without harmful flashback effects, combustion instabilities, or thermal meltdown problems
Applications
- Aircraft engines
- Furnaces (i.e., gas heaters, kiln or furnace burners, utility boiler burners, gas reforming burners, fuel cell processing burners)
- Power (i.e., stationary power plants; hydrogen-air combustion for vehicles)
Similar Results

Advanced Hydrogen and Hydrocarbon Gas Sensors
In conjunction with academia and industry, NASA's Glenn Research Center has developed a range of microelectromechanical systems (MEMS)-based and Silicon Carbide (SiC)-based microsensor technologies that are well-suited for many applications. The suite of technologies includes hydrogen and hydrocarbon leak detection sensors; emissions sensor arrays; and high-temperature contact pads for wire bond connections.
Currently used to protect astronauts on the International Space Station, the hydrogen and leak detection sensors have many Earth-based applications as well. They can function as a single-sensor unit or as part of a complete smart sensor system that includes multiple sensors, signal conditioning, power, and telemetry. The system can comprise sensors for hydrogen, hydrocarbons, oxygen, temperature, and pressure. The emissions sensor array features a gas-sensing structure that detects various combustion emission species (carbon monoxide, carbon dioxide, oxygen, hydrocarbons, and nitrogen oxides) over a wide range of concentrations. In addition, the emissions sensor array remains highly sensitive and stable while providing gas detection at temperatures ranging from 450 to 600°C. These new sensors provide a combination of responsiveness and durability that offers great value for a wide range of applications and industries.

High-Performance, Lightweight, Easy-to-Fabricate Heat Exchanger
Researchers at JPL have developed, built, and tested an innovative heat exchanger that offers reduced thermal expansion, increased structural strength, low pressure drop, and improved thermal performance while lowering the weight associated with typical heat exchangers. This innovation would benefit the commercial thermoelectric generator, aircraft, and industrial processing (i.e., glass, steel, petrochemical, cement, aluminum) industries by improving energy management/efficiency, reducing carbon dioxide emissions, and increasing system durability due to the reduced stress from thermal expansion.
The Problem
Thermoelectric generator systems require high-performance hot-side and cold-side heat exchangers to provide the temperature differential needed to transfer thermal energy while withstanding temperatures up to 650 °C. Because the hot-side heat exchangers must have a high heat flux, they are often made of metals such as stainless steel or Inconel® alloys. Although these materials can operate at high temperatures, resist corrosion, and are chemically stable, they also have several drawbacks: (1) Their lower thermal conductivity negatively affects their thermal performance. (2) Their higher thermal expansion leads to stresses that compromise system structural integrity. (3) Their high mass/volume reduces the power density of generator systems into which they are integrated. As a result, they are difficult to integrate into viable energy recovery systems. They also make the systems unreliable, non-durable, and susceptible to failures caused by thermal-structural expansion.
The Solution
JPL researchers chose to replace the metal in traditional heat exchangers with graphite, which offers an improved conductivity-to-density ratio in thermal applications as well as a low coefficient of thermal expansion. In addition, they used a mini-channel design to further increase thermal performance. Combining more advanced materials with the innovative thermal design has yielded significant improvements in performance. For example, a 200-cm3, 128-g version of JPL's exchanger successfully transported 1,100 W from exhaust at nearly 550 °C with approximately 20 W/cm2 thermal flux and a pressure drop of only 0.066 psi.
JPL's technology combines lightweight, high-strength graphite material with a mini-channel design that offers high thermal performance. Further development and testing are underway.
Inconel is a registered trademark of Special Materials Corporation.

Low-Temperature Oxidation/
Reduction Catalysts
The low-temperature oxidation catalyst technology employs a novel catalyst formulation, termed platinized tin oxide (Pt/SnOx). The catalysts can be used on silica gel and cordierite catalyst supports, and the latest developments provide sprayable formulations for use on a range of support types and shapes. Originally developed for removal of CO, the catalyst has also proven effective for removal of formaldehyde and other lightweight hydrocarbons.
NASA researchers have also extended the capability to include reduction of NOx as well as developed advanced chemistries that stabilized the catalyst for automotive catalytic converters via the engineered addition of other functional components. These catalyst formulations operate at elevated temperatures and have performed above the EPA exhaust standards for well beyond 25,000 miles. In addition, the catalyst can be used in diesel engines because of its ability to operate over an increased temperature range.
For use as a gas sensor, the technology takes advantage of the exothermic nature of the catalytic reaction to detect formaldehyde, CO, or hydrocarbons, with the heat being produced proportional to the amount of analyte present.

High-Temperature Single Crystal Preloader
For extremely high-temperature sealing applications, Glenn researchers have devised novel methods for fabricating single-crystal preloaders. NASA's high-temperature preloaders consist of investment cast or machined parts that are fabricated in various configurations from single crystal superalloys. Machined preloaders include a variety of spring configurations, compressed axially or radially, fabricated from single crystal slabs. Before machining, the slabs are carefully oriented in a special goniometer using x-diffraction techniques. This helps to maintain proper crystal orientation relative to the machined part and the applied loads. For more complex geometry components which cannot be easily and economically machined, an investment casting approach would be used. Complex preloader geometries include wire coil springs of various configurations. These single crystal preloaders would be designed with the appropriate stiffness for the intended thermal barrier/seal application and placed underneath, or integrated within, the seal/barrier. At extrememly high temperature, the preload device keeps the seal/barrier mated against the opposing surface as the gap between the two surfaces changes, maintaining contact between surfaces and preventing convective heat transfer.

Coil-On-Plug Igniter for Reliable Engine Starts
Spark-ignition devices have proven to be a high-reliability option for LOX/LCH4 ignition during development of the Integrated Cryogenic Propulsion Test Article (ICPTA) main and reaction control engines (RCEs); however, issues including spark plug durability (ceramic cracking) and corona discharge during simulated altitude testing have been observed, contributing to degraded spark output and no-light engine-start conditions. Innovators discovered that ignition system reliability could be improved and weight reduced by eliminating the traditional coil and spark plug wire. To achieve this result, engineers made the innovation by modifying an automotive coil-on-plug igniter to provide new high sparking energies at the point of combustion using low supply voltages. The coil was modified by vacuum-potting it into a threaded interface that mounts into existing spark plug ports on the ICPTA main engine and the RCEs. Engineers fabricated custom electrode tips that were thread-mounted into the potted coil body. Epoxy insulation was chosen with high dielectric strength to maintain insulation between the electrode and threaded adapter. Vacuum potting successfully prevented pressure or vacuum leakage into the coil body and maintained spark energy and location at the electrode tip. Successful hot-fire ignition was observed at sea-level, altitude, and thermal-vacuum for both ICPTA RCE and main engine igniters down to 10^-3 torr, which approaches the vacuum of cislunar space.
This technology is at technology readiness level (TRL) 7 (system prototype demonstration in an operational environment), and the related patent is now available to license. Please note that NASA does not manufacture products itself for commercial sale.