NanoWire Glass Switch for Radio Frequency
communications
NanoWire Glass Switch for Radio Frequency (LEW-TOPS-24)
Invisible switch outlasts MEMS switches for RF applications
Overview
NASA's Glenn Research Center has developed nanoionics-based radio frequency (RF) switches for use in devices that rely on low power RF transmissions, such as automotive systems, RFID technology, and smart phones. These groundbreaking nanoionic switches operate at speeds of semiconductor switches and are more reliable than microelectromechanical systems (MEMS) switches, while retaining the superior RF performance and low power consumption found in MEMS all without the need for higher electrical voltages. In this new process, metals are photo-dissolved into a chalcogenide glass and packaged with electrodes and a substrate to form a switch. Since the nanoionics-based switch is electrochemical in nature, it has certain advantages over switches that are mechanically based, including non-volatility, lack of moving parts that can fail, ease and efficiency of activation, and ease of fabrication. This innovative device has the potential to replace MEMS and semiconductors in a wide range of switching systems, including rectifying antennas (rectennas) and other RF antenna arrays.
The Technology
The nanoionic-based switches developed by NASA's Glenn Research Center exploit the properties of some amorphous materials that can incorporate relatively large amounts of metal and behave as solid electrolytes. As with liquid electrolytes found in lead-acid batteries, for example, solid electrolytes consist of mobile ions which undergo oxidation/reduction reactions at the anode and cathode of the system. The ionic conductivity of such a material can be of the same order of magnitude as the electronic conductivity of a semiconductor but without the drawbacks of an electromechanical device.
In the nanoionic switch, ions are formed at an anode and migrate into the solid electrolyte, while electrons are injected from a cathode, thereby causing the growth of metal nanowires through the electrolyte from the cathode to the corresponding anode when a positive DC bias is applied. Once a nanowire has grown sufficiently to form an electrically conductive path between the electrodes, the switch is closed and no electric power is needed to maintain the connection, unlike in a MEMS or semiconductor-based switch. Moreover, the process of making the connection can easily be reversed by applying a negative bias, causing the wires to ungrow and the switch to open. Thus, NASA's state-of-the-art device is a reversible electrochemical switch that can have geometric features as small as nanometers. The process time for making or breaking the connection is very brief -- about a nanosecond. In addition, this nanoionic material can be deposited in such a way to form multilayer control circuits, which has the potential to minimize circuit footprints, reduce overall circuit losses, and provide unprecedented ease of integration.
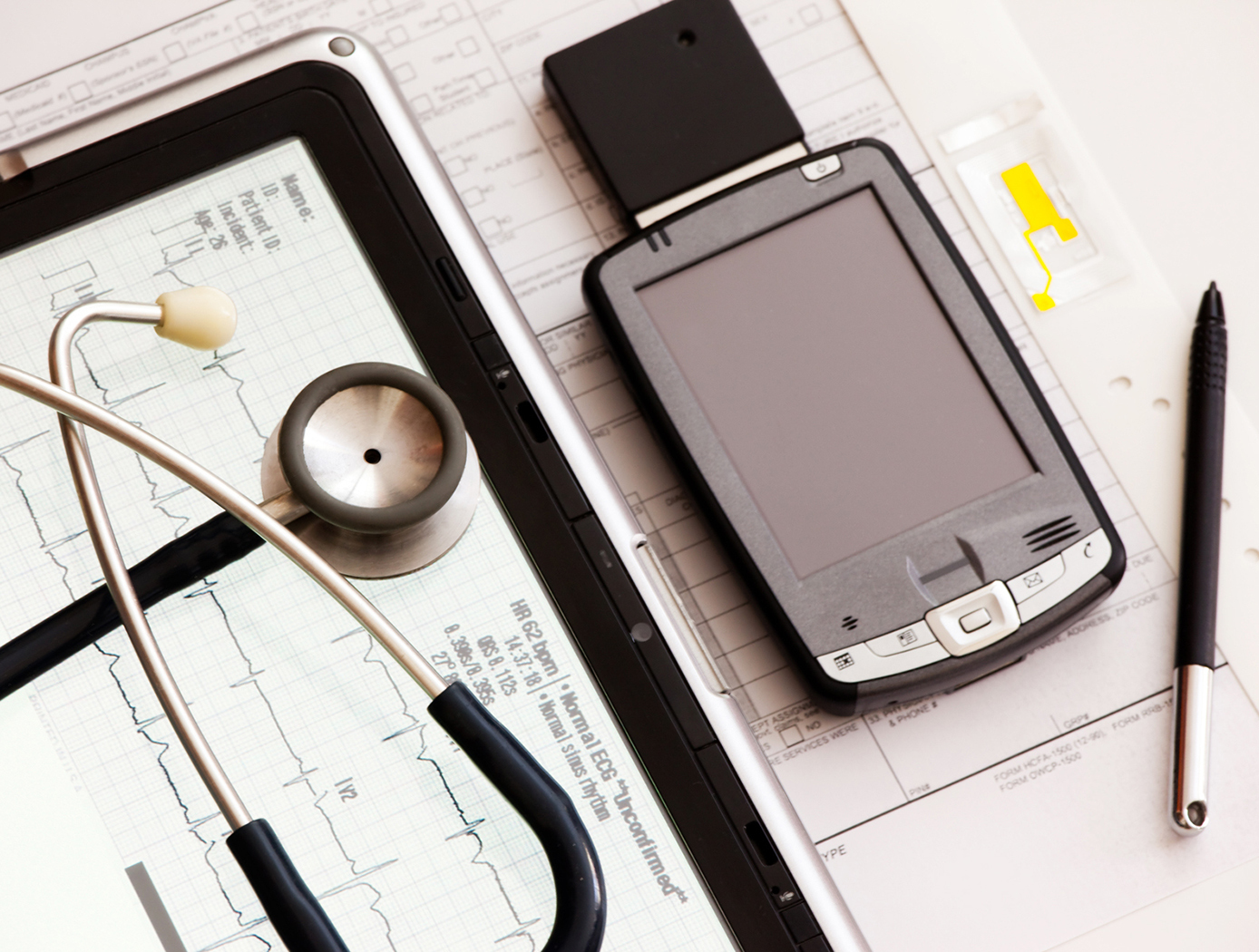
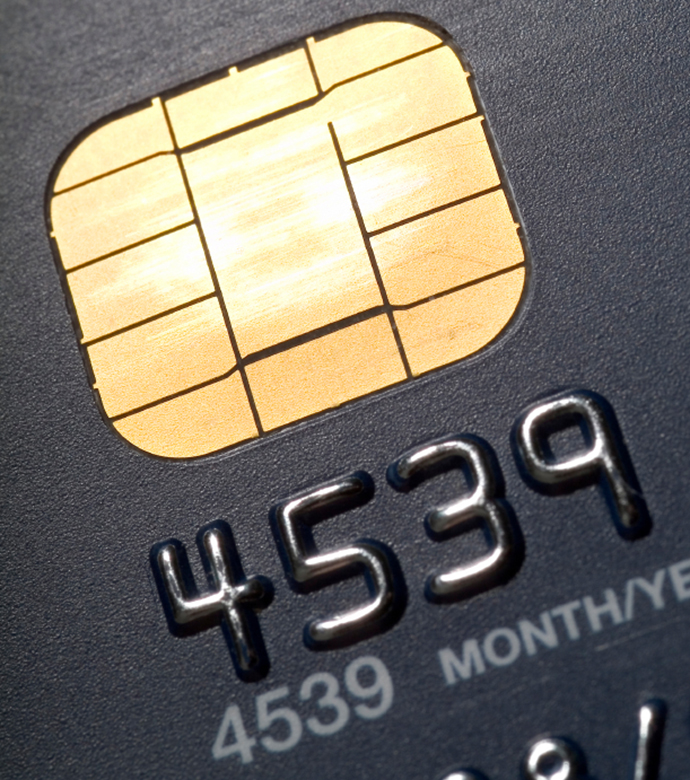
Benefits
- Low power: Requires 1 volt, as opposed to 50 to 60 volts for microelectromechanical systems (MEMS)
- Lower cost: Less expensive than MEMS and uses conventional integrated-circuit fabrication techniques, with as few as five processing steps
- Simpler: Nonvolatilemeaning no power is required to maintain a particular state (e.g., ON or OFF)
- Faster: Comparable in speed to solid-state electronics
- More rugged and reliable: Maintains superior performance as compared to a mechanical connection, with no moving parts and longer mean time before failure
Applications
- Conformal antennas for automotive collision avoidance systems and navigation; e.g., Global Positioning Systems (GPS)
- Phased arrays for communications and data storage, especially in broadcasting and radar (mobile device data streaming, military, satellite television)
- Rectennas in smart keys, safety devices, and other radio-frequency identification (RFID)-based devices
- Sensing and diagnostic systems (health care, oil & gas, utilities)
Tags:
|
Similar Results

Polymer Nanofiber-Based Reversible Nano-Switch/Sensor Schottky Diode Device
Glenn's innovative nanoSSSD device includes a doped semiconducting substrate, an insulating layer deposited on the substrate, an electrode formed on the insulating layer, and at least one polymer nanofiber deposited on the electrode. The deposited nanofiber provides an electrical connection between the electrode and the substrate, serving as the electro-active element in the device. The nanofiber is generally composed of a customized polymer (e.g., polyaniline) that is extremely sensitive to the adsorption and desorption of a single gas molecule. As gas molecules are adsorbed and desorbed, the resistivity of the customized polymer also changes, providing its sensing capacity. When the nanoSSSD device senses a selected gaseous species, the switching portion of the device automatically actuates, sending a signal to the control component. This control component activates the output (warning) device. In addition to its ability to detect harmful gases (including ammonia, hydrogen, hydrocarbons, nitrogen oxides, carbon monoxide, and carbon dioxide), Glenn's device can also feature conducting polymers that are sensitive to UV radiation. Glenn's nanoSSSD technology has great commercial potential, particularly in situations where frequent replacement of the switch/sensor is impractical.

Inexpensive Microsensor Fabrication Process
Because chemical sensors are used in many aspects of space missions, NASA researchers are continually developing ever smaller and more robust sensors that can be manufactured inexpensively and in high quantities; e.g., in batches. Glenn has developed a way to inexpensively fabricate microsensors using a sacrificial template approach. A nanostructure, such as a carbon nanotube, serves as a template, which can then be coated with a high-temperature oxide material. The carbon nanotube can be burned off, or sacrificed, leaving only the metal oxide. The resulting structure provides the unique morphology and properties of the carbon nanotube, which are advantageous for sensing, along with the material durability and high-temperature sensing capabilities of the metal oxide. This technique increases the surface area available for sensing because both the interior and exterior of the resulting microsensor can be used for gas detection, significantly increasing performance.
The fabrication of these microsensors includes three major steps: (1) synthesis of the porous metal or metal oxide nanostructures using a sacrificial template, (2) deposition of the electrodes onto alumina substrates, and (3) alignment of the nanostructures between the electrodes. The invention has been demonstrated for methane detection at room temperature (using tin oxide, with carbon nanotubes as the sacrificial template). The microsensor offers low power consumption (no heating required), compact size, extremely low cost, and simple batch-fabrication.

Portable Compact Thermionic Power Cell
This compact thermionic cell (CTI) technology can be manufactured efficiently and economically using existing semiconductor fabrication technology. Its design consists of a top electron collector, separated by a vacuum gap from an electron emitter adjacent to the heat source, a thin plate of 238Pu enclosed by a thin-film insulator to protect the emitter and collector layers from overheating by the 238Pu. For a smart phone battery size, the invented compact thermionic (CTI) cell requires about 5 g maximum of 238Pu. Such small quantities are more readily available and producible, and could be reused for recycling when the CTI cell is dismantled. The emitter surface is topologically modified to have array of spikes, achievable using current semiconductor microfabrication technology. Various other geometries of emitter plates may also be used, such as an array of ridges. The smaller the emitter tips, the higher the voltage concentration.

Low-Power Charged Particle Detector
Conventional scintillation radiation detectors make use of materials that emit light when hit by ionizing radiation. These large, bulky scintillators range anywhere from 6 inches to 6 feet in size. They are attached to glass photomultiplier tubes (PMTs), which are fragile, require high voltages, and are extremely sensitive to temperature changes. Conventional detectors also include a wave shifter (a material with a dopant to re-emit the scintillator light to match the sensitivity of the PMT or photodiode) which reduces efficiency and introduces unwanted weight and bulk. Glenn's particle counter overcomes these challenges. A prototype was constructed from off-the-shelf components, and features a small scintillator (less than six inches long) and a low-voltage, UV-sensitive, wide-bandgap photodiode as the detector. Careful matching of the properties of the light emitted from the scintillator, and tailoring the sensitivity of the photodiode eliminates the need for a PMT and a wave shifter, not only saving space, weight, and power but also eliminating potential failure modes. By using wide-bandgap detectors, it can operate in changing temperature, vibration, pressure, and gravity conditions without need for a temperature compensation system. Built from solid-state components, Glenn's device is compact and robust.

Solar Powered Carbon Dioxide (CO2) Conversion
This technology consists of a photoelectrochemical cell composed of thin metal oxide films. It uses sunlight (primarily the ultraviolet (UV), visible and Infrared (IR) portions)) and inexpensive titanium dioxide composites to perform the reaction. The device can be used to capture carbon dioxide produced in industrial processes before it is emitted to the atmosphere and convert it to a useful fuel such as methane. These devices can be deployed to the commercial market with low manufacturing and materials costs. They can be made extremely compact and efficient and used in sensor and detector applications.