Metal Oxide-Vertical Graphene Hybrid Supercapacitors
electrical and electronics
Metal Oxide-Vertical Graphene Hybrid Supercapacitors (TOP2-223)
Hybrid supercapacitor system utilizing vertical graphene
Overview
NASA has developed a novel hybrid supercapacitor system utilizing vertical graphene as an electrode material grown directly on collector metals using a plasma enhanced chemical vapor deposition. Supercapacitors are an alternative to batteries for energy storage, offering high power density and rapid charging time. Nanomaterials such as carbon nanotubes and graphene offer high surface area and porosity to construct the electrodes. Vertical graphene grown directly on a collector metal substrate enables construction of a supercapacitor. The key to the hybrid supercapacitor technology is the growth of vertical graphene directly on to an inexpensive metal substrate without the use of bulk graphene, catalysts, or binders, resulting in increased power density. Adding the metal oxide or electrically conducting polymer to the vertical graphene adds redox (reduction and oxidation) capacitance, thus increasing the overall performance of the device.
The Technology
The electrodes are soaked in electrolyte, separated by a separator membrane and packaged into a cell assembly to form an electrochemical double layer supercapacitor. Its capacitance can be enhanced by a redox capacitance contribution through additional metal oxide to the porous structure of vertical graphene or coating the vertical graphene with an electrically conducting polymer. Vertical graphene offers high surface area and porosity and does not necessarily have to be grown in a single layer and can consist of two to ten layers. A variety of collector metals can be used, such as silicon, nickel, titanium, copper, germanium, tungsten, tantalum, molybdenum, & stainless steel.
Supercapacitors are superior to batteries in that they can provide high power density (in units of kw/kg) and the ability to charge and discharge in a matter of seconds. Aside from its excellent power density, a supercapacitor also has a longer life cycle and can undergo many more charging sequences in its lifespan than batteries. This long life cycle means that supercapacitors last for longer periods of times, which alleviates environmental concerns associated with the disposal of batteries.
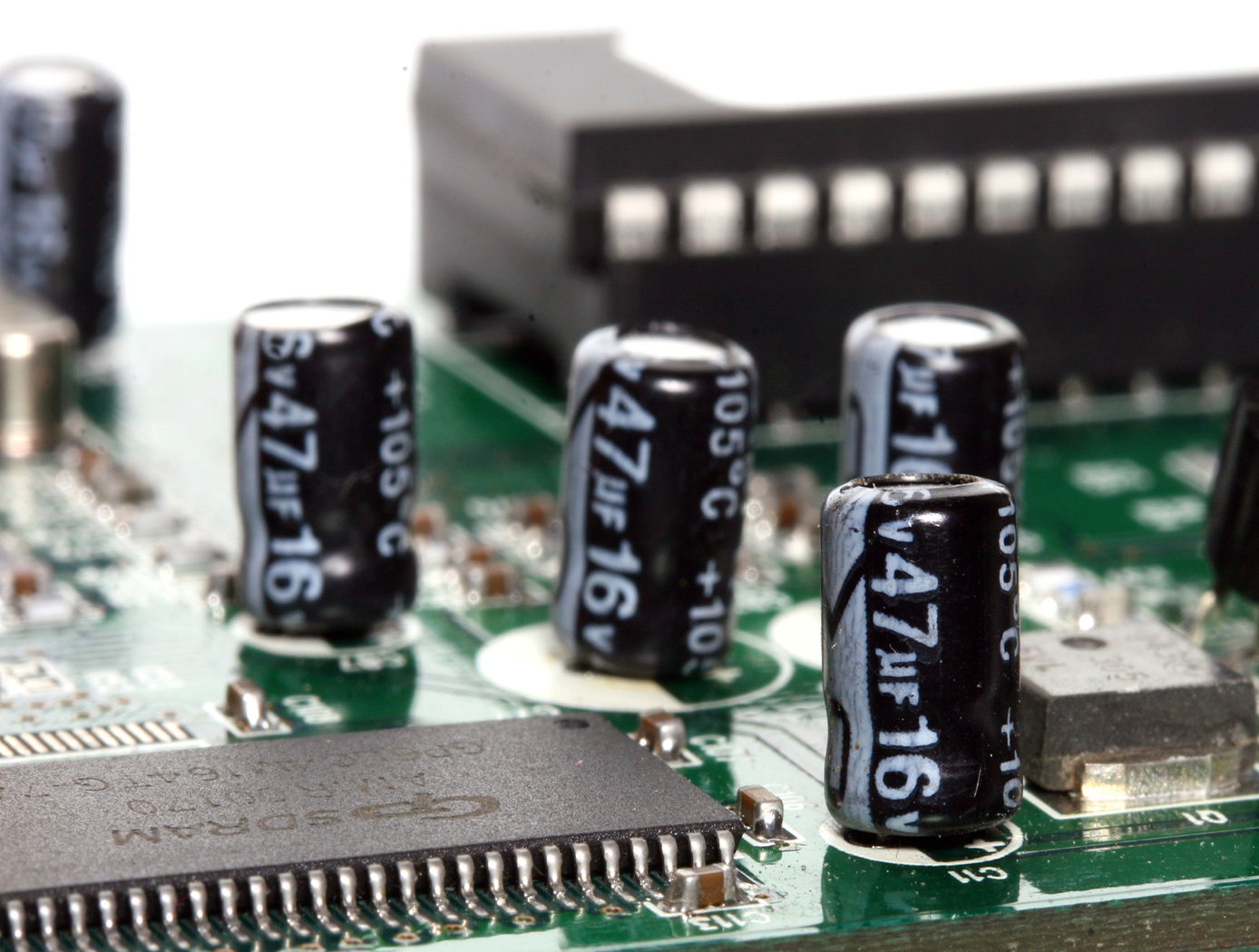
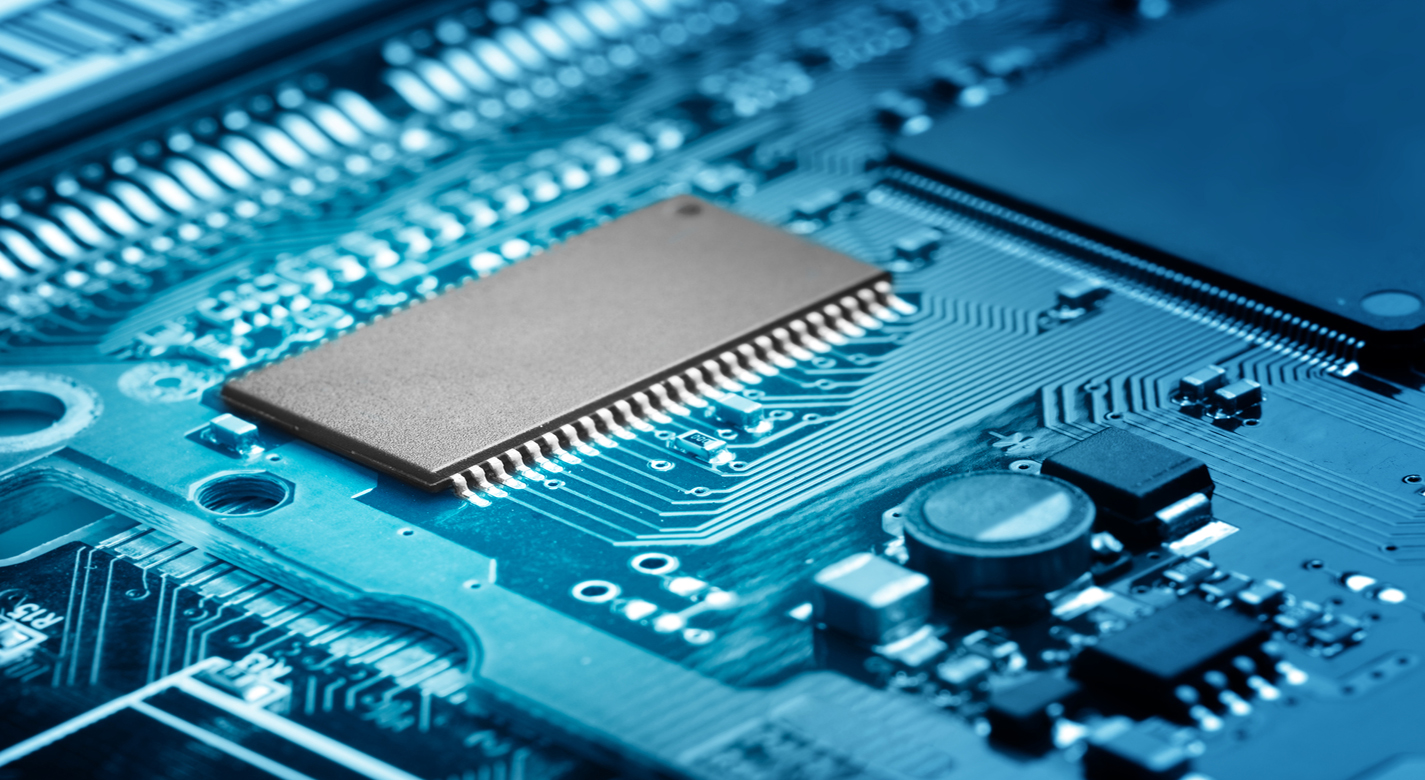
Benefits
- High power density
- Rapid charging time
- No catalyst or binders, thus reduced interfacial resistance
- Long life cycle
- Hybrid supercapacitor by adding metal oxide or electrically conducting polymer to vertical graphene
Applications
- Electric Automobile power sources
- Renewable energy storage
- Energy and Environmental Design
- Consumer electronic products
- Power delivery solutions for forklifts, robots, buses, trolleys, light rail
- Computer memory back-up devices
Tags:
|
Similar Results

Holey Carbon Allotropes
This invention is for scalable methods that allows preparation of bulk quantities of holey nanocarbons with holes ranging from a few to over 100 nm in diameter. The first method uses metal particles as a catalyst (silver, copper, e.g.) and offers a wider range of hole diameter. The second method is free of catalysts altogether and offers more rapid processing in a single step with minimal product work-up requirements and does not require solvents, catalysts, flammable gases, additional chemical agents, or electrolysis. The process requires only commercially available materials and standard laboratory equipment; and, it is scalable. Properties that can be controlled include: surface area, pore volume, mechanical properties, electrical conductivity, and thermal conductivity.

Novel, Solid-State Hybrid Ultracapacitor Battery
The subject technology is an extension of closely related, solid-state ultracapacitor innovations by the same team of inventors. The primary distinction for this specific technology is the addition of co-dopants to affect the dielectric behavior of the barium titanatebased perovskite materials. These co-dopants include lanthanum and other rare earths as well as hydroxyl ions. The materials are processed at the nano scale, and are subjected to carefully designed thermal treatments as well.
The presence of the hydroxyl ions has been shown to provide several orders of magnitude increase in the capacitance of the dielectric material. Additionally, these high capacitance values are obtained at relatively low voltages found in current consumer and industrial electronics.
The capacitors tested to date are simple, single-layer devices. Ultimately, a range of manufacturing methods are possible for making commercial devices. Features of the technology enable manufacturing via traditional thick-film processing methods widely used in the capacitor industry, or via advanced printing methods for state-of-the-art printed electronics.
Future efforts will be made to advance the manufacturing and packaging processes to increase device energy density, including multilayer devices and packages

Carbon Bipolar Membranes for Solid-State Batteries
In traditional batteries with liquid electrolytes, e.g., lithium-ion, each battery cell must be individually sealed, packaged, and electrically connected to other cells in the pack. The cells in solid-state batteries on the other hand may be stacked on top of one another with only a separation layer in between, called a bipolar plate. These bipolar plates or membranes if thin enough must be electrochemically inert to the electrode and electrolyte materials while providing electrical connectivity between the individual cells.
Here, NASA has combined advances in the preparation of carbon nanomaterials and solid-state batteries to create extremely lightweight bipolar plates and membranes. These bipolar membranes will enable high energy density solid-state batteries unachievable with typical bipolar plate materials like stainless steel, aluminum, aluminum-copper, or conductive ceramics. The carbon bipolar membranes may be fabricated in multiple ways including but not limited to directly compressing carbon powders onto an electrode-electrolyte stack or separately making a film of the carbon material and dry pressing the film between other battery layers. The new bipolar membranes have been demonstrated in high energy density solid-state batteries in coin and pouch cells.
The carbon bipolar membranes are at technology readiness level TRL-4 (Component and or breadboard validation in laboratory environment)and are available for patent licensing.

Room temperature oxygen sensors
NASA Ames has developed very small-sized oxygen sensors made of a graphene and titanium dioxide (TiO2) hybrid material. With ultraviolet (UV) illumination, these sensors are capable of detecting oxygen (O2) gas at room temperature and at ambient pressure. The sensors are able to detect oxygen at concentrations ranging from about 0.2% to about 10% by volume under 365nm UV light, and at concentrations ranging from 0.4% to 20% by volume under short wave 254nm UV light. These sensors have fast response and recovery times and can also be used to detect ozone.
This unique room temperature O2 sensor provides significant advantages in O2 sensing applications, especially those applications where high operating temperature requirements cannot be met, or would result in inefficient manufacturing processes. Since graphene is not intrinsically responsive to O2, and TiO2 is not responsive to oxygen at room temperature, the materials are first synthesized as a hybrid material. The synthesized graphene- TiO2 hybrid material is then ultrasonicated and then drop-casted onto a series of Interdigitated Electrodes (IDE) to form the sensors.
Ultrasonication ensures effective charge transfer at the graphene- TiO2 interphase. The graphene and the titanium dioxide may be present in the composite material in different ratios to ensure optimal oxygen detection. It is the combination of graphene with TiO2 that yields a semiconducting material capable of O2 sensing at room-temperature operation.

Cryogenic Flux Capacitor
Storage and transfer of fluid commodities such as oxygen, hydrogen, natural gas, nitrogen, argon, etc. is an absolute necessity in virtually every industry on Earth. These fluids are typically contained in one of two ways; as low pressure, cryogenic liquids, or as a high pressure gases. Energy storage is not useful unless the energy can be practically obtained ("un-stored") as needed. Here the goal is to store as many fluid molecules as possible in the smallest, lightest weight volume possible; and to supply ("un-store") those molecules on demand as needed in the end-use application. The CFC concept addresses this dual storage/usage problem with an elegant charging/discharging design approach.
The CFC's packaging is ingeniously designed, tightly packing aerogel composite materials within a container allows for a greater amount of storage media to be packed densely and strategically. An integrated conductive membrane also acts as a highly effective heat exchanger that easily distributes heat through the entire container to discharge the CFC quickly, it can also be interfaced to a cooling source for convenient system charging; this feature also allows the fluid to easily saturate the container for fast charging. Additionally, the unit can be charged either with cryogenic liquid or from an ambient temperature gas supply, depending on the desired manner of refrigeration. Finally, the heater integration system offers two promising methods, both of which have been fabricated and tested, to evenly distribute heat throughout the entire core, both axially and radially.
NASA engineers also applied the CFC to a Cryogenic Oxygen Storage Module to store oxygen in solid-state form and deliver it as a gas to an end-use environmental control and/or life support system. The Module can scrub out nuisance or containment gases such as carbon dioxide and/or water vapor in conjunction with supplying oxygen, forming a synergistic system when used in a closed-loop application. The combination of these capabilities to work simultaneously may allow for reduced system volume, mass, complexity, and cost of a breathing device.