Search
Sensors

Quantum Cascade Laser Source and Transceiver
The QCL source addresses the challenges of inefficiency, high power consumption, and bulky designs typically associated with existing solutions. It is fabricated with 80 to 100 alternating layers of semiconductor materials, each layer only a few microns thick. These layers create a cascade effect that amplifies terahertz-energy photon generation while consuming significantly less voltage. To mitigate the natural beam dissipation of QCLs, the source is integrated with a waveguide and thin optical antenna, reducing signal loss by 50%. Additionally, the waveguide employs a flared design with a diagonal feed horn, achieving high modal confinement and increasing beam coupling efficiency to 82%, compared to 37% in conventional setups. This compact design, smaller than a U.S. quarter, fits within payload constraints and enables high-powered terahertz beams for precise spectroscopic measurements.
The terahertz transceiver enhances measurement precision by integrating two back-to-back hybrid couplers and Schottky diodes as detectors, providing a 35 dB dynamic range. Operating in the 2.0–3.2 THz frequency range, the transceiver is optimized for versatility across astrophysics, heliophysics, and planetary science applications. It seamlessly couples the QCL-generated signal onto the waveguide, ensuring stable and accurate spectroscopic data collection. This compact and energy-efficient transceiver delivers exceptional sensitivity, enabling it to analyze planetary materials, atmospheric components, and interstellar phenomena with unmatched resolution.
With its compact, tunable design and high spectral resolution, the QCL source and transceiver represents a significant advancement for remote sensing and planetary surface characterization, offering a versatile solution for both NASA and commercial applications. The QCL system is at technology readiness level (TRL) 4 (component and/or breadboard validation in lab) and is available for patent licensing.
Electrical and Electronics

Broadband Metamaterial Termination for Planar Superconducting Transmission Line Circuits
The broadband metamaterial termination for use in planar superconducting transmission lines has been successfully demonstrated in CLASS circuit structures as an effective termination. This metamaterial implementation is fully compatible with microfabrication techniques commonly used for microwave circuitry, and its response is insensitive to geometric tolerances, material properties, and interface details of conductive elements in device fabrication. In the context of far-infrared imaging, polarimetric, and superconducting integral field unit (IFU) spectrometer arrays for astrophysics, this strategy leads to higher performance, increased device yield, and greater overall circuit density.
The metamaterial termination achieves a broadband absorption response with lower reflectance in a smaller physical footprint compared to existing adiabatic structures. This absorption response demonstrates significantly lower sensitivity to fabrication tolerances, material properties, and modeling assumptions than previous designs. These characteristics are critical for cryogenic applications, but the termination can also enhance the performance of room-temperature planar transmission line structures used in microwave engineering. The termination is realized as a lossy stepped impedance transition between Nb and PdAu, which reduces the total meander length, device footprint, and sensitivity to detailed implementation.
This broadband metamaterial termination is applicable in superconducting technologies, including quantum communications, computing, and sensors. It has reached Technology Readiness Level (TRL) 7 (technology demonstrated in an operational environment) and is now available for patent licensing.
Communications

Boosting Quantum Communication Efficiency
The technology consists of an array of quantum photon sources connected via a sophisticated switching network. This system is designed to produce single pairs of entangled photons at a high rate while actively suppressing the generation of multiple pairs. The key innovation lies in its ability to detect and eliminate instances where two or more entangled photon pairs are generated, effectively reducing noise in the quantum system.
The technology operates by providing a heralding pulse that notifies the external system of successful entangled photon generation. When multiple pairs are detected, they are prevented from entering the rest of the system, thereby maintaining the integrity of the quantum information.
By combining multiple single-photon sources through its switching network, the technology not only reduces noise but also increases the overall single photon pair generation rate. This dual approach of noise reduction and increased generation efficiency improves qubit transmission rates, potentially by a factor of 10 to 100 over current methods.
While still in the early stages of development, the source array represents a significant advancement in quantum communication systems. It addresses the critical need for high-fidelity entangled photon sources, which are essential for various quantum applications, including entangling sensor networks, quantum computer networks, and quantum key distribution for secure communications.
As quantum technologies continue to evolve, this source array technology positions itself as a crucial component in the development of large-scale, efficient quantum networks, offering a solution to one of the fundamental challenges in quantum information transmission.
communications
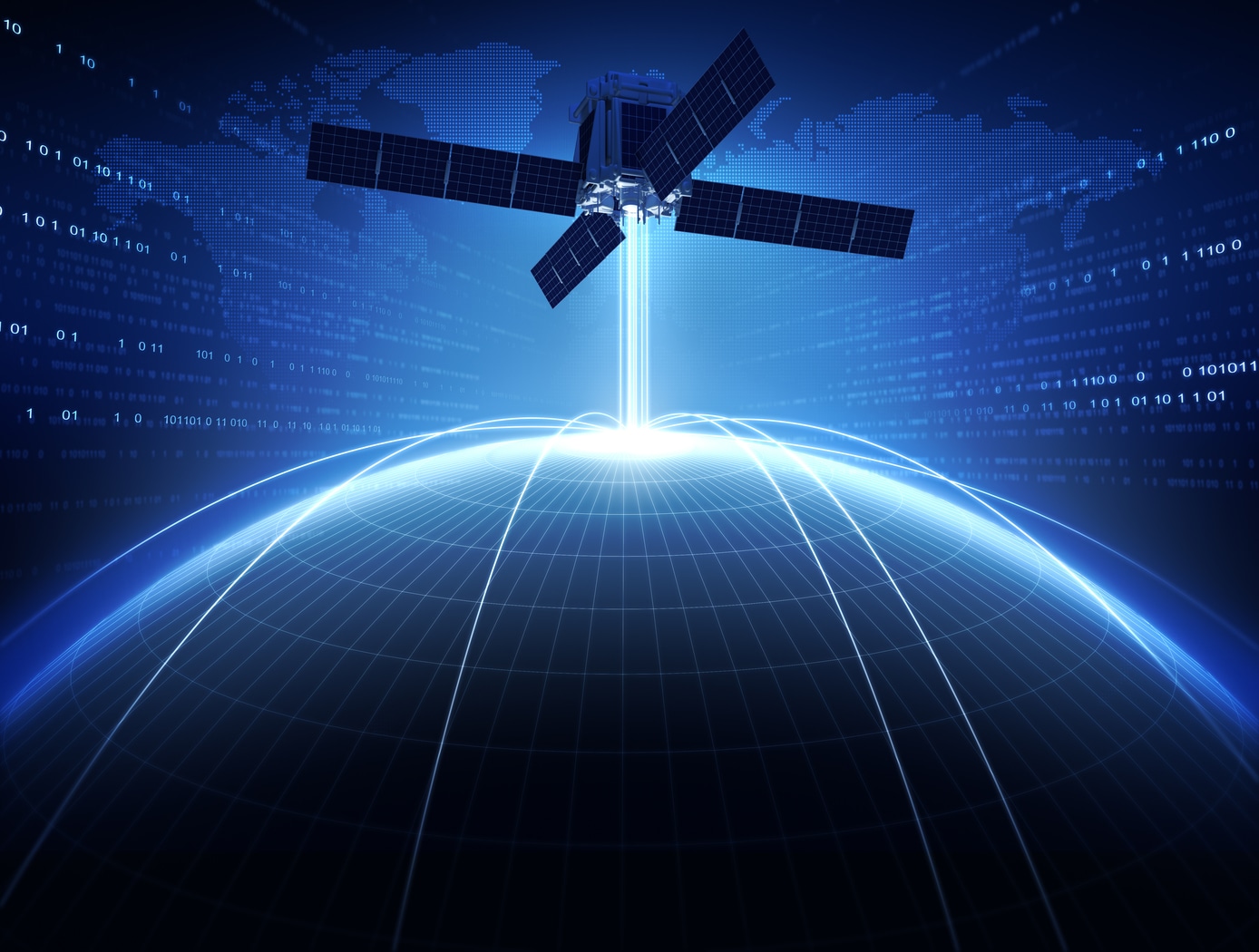
Optical De-Multiplexing Method for QKD Encryption
Classical laser communication gimbals are coupled to 105um multimodal receiving fibers for the high-power transmission of data, fine pointing, and tracking. These fibers cannot be used in free space optical communication applications using Quantum Key Distribution (QKD) since polarization state information encoded by QKD photons is not retained. To accommodate low energy QKD photons and high energy data streams necessary for encryption of optical links, the inventor adopted a space-and-wave (SAW) division de-multiplexing approach.
The SAW division method uses a double clad fiber with a 9um core and a 105um 1st cladding. This arrangement captures 1590nm wavelength QKD photons in the core channel and a 1555.75nm wavelength data channel in the 1st cladding. By defining wavelength separation between 30-40nm, a single focusing lens can be used to focus only one wavelength to a diffraction limited spot (see figures included). Using this method, a QKD channel is focused to a diffraction limited spot on the 9um core of the double clad fiber. The chosen wavelength separation generates a defocused diffraction pattern with a hollow center, and with remaining optical power in concentric rings outside of the 9um core, yet inside the 105um core. The QKD signal is directed into the 9um core, and the data channel is coupled into the 105um secondary core for traditional data demodulation.