Optical De-Multiplexing Method for QKD Encryption
communications
Optical De-Multiplexing Method for QKD Encryption (LEW-TOPS-163)
Space-and-wave division for quantum key distribution photon detection
Overview
NASA inventors have developed a space-and-wave (SAW) division method to de-multiplex low energy quantum key distribution (QKD) photons from high energy free space optical transmissions. The method exploits available double-clad fiber optic cable with 9um core fiber diameter and 105um 1st cladding outer diameter. By optimizing wavelengths used for QKD photon and data transmission (wave division), a focusing lens can be used to create a diffraction pattern that focuses QKD photons on the core and the higher energy signal onto the 1st cladding (spatial division). The SAW method enables de-multiplexing of simultaneously transmitted optical data and built-in photonic QKD encryption keys. The method was developed by NASA to send and receive encryption keys using weak coherent pulsed light rather than entangled photons; it can be applied to the encryption of any free space optical communications.
The Technology
Classical laser communication gimbals are coupled to 105um multimodal receiving fibers for the high-power transmission of data, fine pointing, and tracking. These fibers cannot be used in free space optical communication applications using Quantum Key Distribution (QKD) since polarization state information encoded by QKD photons is not retained. To accommodate low energy QKD photons and high energy data streams necessary for encryption of optical links, the inventor adopted a space-and-wave (SAW) division de-multiplexing approach.
The SAW division method uses a double clad fiber with a 9um core and a 105um 1st cladding. This arrangement captures 1590nm wavelength QKD photons in the core channel and a 1555.75nm wavelength data channel in the 1st cladding. By defining wavelength separation between 30-40nm, a single focusing lens can be used to focus only one wavelength to a diffraction limited spot (see figures included). Using this method, a QKD channel is focused to a diffraction limited spot on the 9um core of the double clad fiber. The chosen wavelength separation generates a defocused diffraction pattern with a hollow center, and with remaining optical power in concentric rings outside of the 9um core, yet inside the 105um core. The QKD signal is directed into the 9um core, and the data channel is coupled into the 105um secondary core for traditional data demodulation.
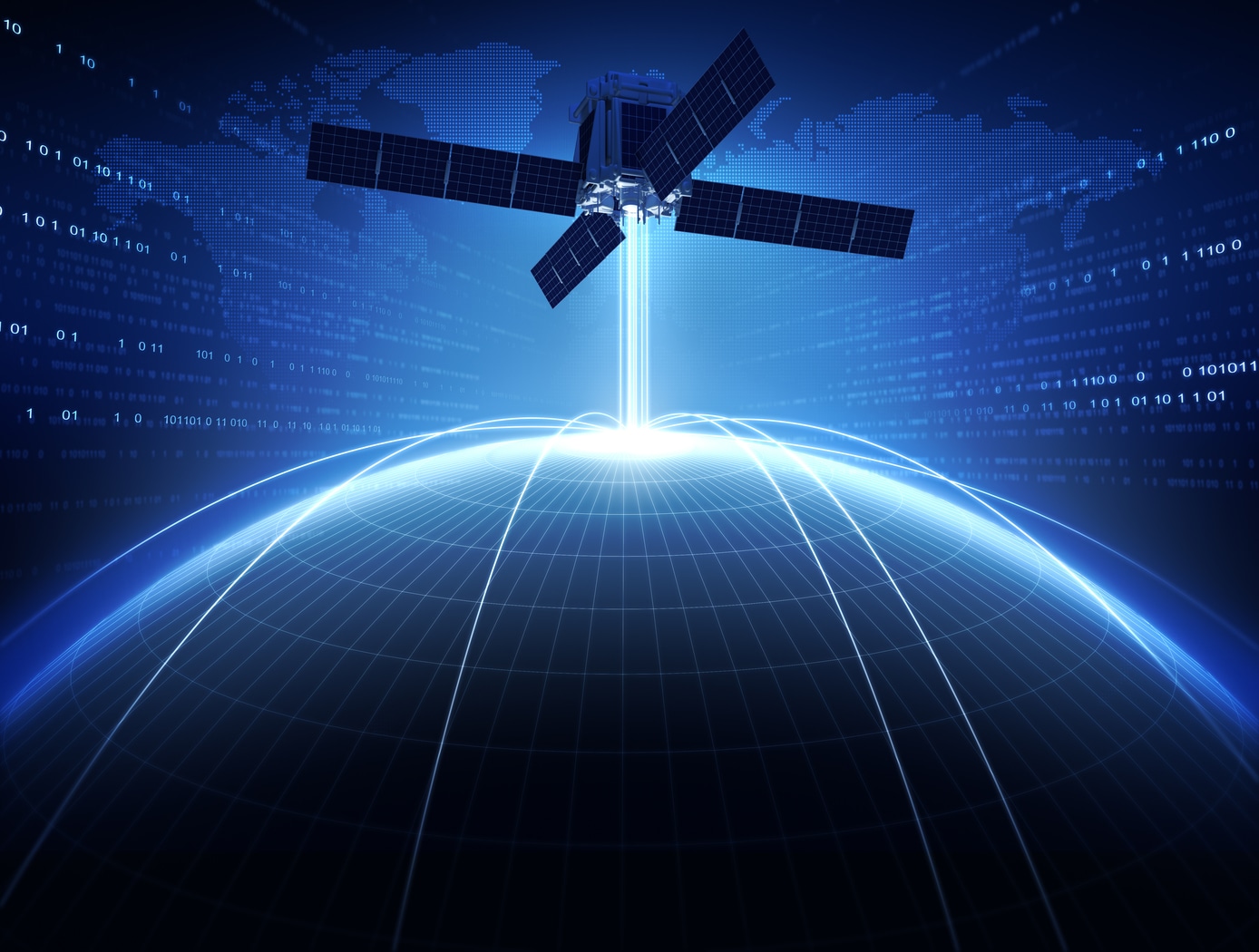
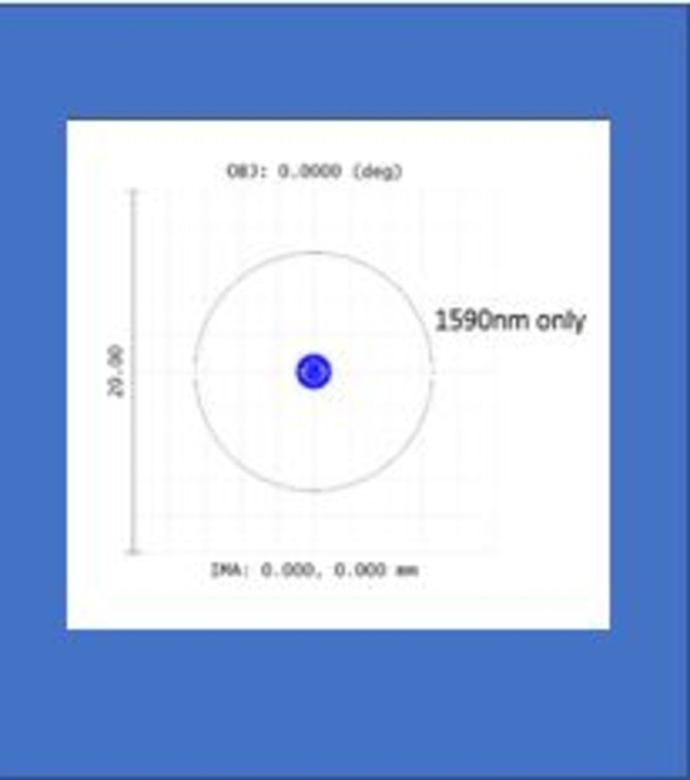
Benefits
- Enabling: allows practical application of QKD to free space optical communication
- Universal: can be applied to the encryption of any free space optical communication
- Expandability: additional channels can be included for timing and synchronization duties
- Hardware compatibility: leverages commercially available double-clad fiber
Applications
- Telecommunications: QKD-encrypted optical data links (satellite-to-ground, satellite-to-satellite, aircraft-to-ground, etc.)
Tags:
|
Similar Results

Secure Optical Quantum Communications
In the prior art, the systems that produced photon pairs took up a great deal of space on a laboratory table, weighed several hundred pounds, consumed tens of kilowatts of electrical power, and required cooling water. These limitations greatly restricted the utility of quantum communications systems, which rely on these photon pairs. To address this issue, Glenn's innovators developed a novel system that uses a laser source, a pair of nonlinear crystals in optical contact with each other, and a fiber coupling point configured to receive a pair of single mode fibers. Pairs of polarization-entangled photons are produced through spontaneous parametric down conversion of the laser beam and provided to the fiber coupling point. The optical signal is coded at the transmitter by modulating the inter-beam delay time between pairs of entangled photons. The inter-beam delay will determine whether the photon pairs are absorbed by a fluorescer in the receiver. When absorbed, the photon pairs cause a fluorescent optical emission that a photon detector identifies. One advantage of this system is that it eliminates the need for a coincidence counter to realize the entanglement-based secure optical communications, because the absorber acts as a coincidence counter for entangled photon pairs. In addition, this modulation spectroscopy technique is ultra-secure since the delay times are very short (femtoseconds) and unresolvable by conventional photon detectors. Finally, the system uses solid-state, monolithic construction that allows for cost-effective batch-manufacturing techniques. This technology represents a significant breakthrough in the fields of communications, optics, cryptography, and surveillance.

Boosting Quantum Communication Efficiency
The technology consists of an array of quantum photon sources connected via a sophisticated switching network. This system is designed to produce single pairs of entangled photons at a high rate while actively suppressing the generation of multiple pairs. The key innovation lies in its ability to detect and eliminate instances where two or more entangled photon pairs are generated, effectively reducing noise in the quantum system.
The technology operates by providing a heralding pulse that notifies the external system of successful entangled photon generation. When multiple pairs are detected, they are prevented from entering the rest of the system, thereby maintaining the integrity of the quantum information.
By combining multiple single-photon sources through its switching network, the technology not only reduces noise but also increases the overall single photon pair generation rate. This dual approach of noise reduction and increased generation efficiency improves qubit transmission rates, potentially by a factor of 10 to 100 over current methods.
While still in the early stages of development, the source array represents a significant advancement in quantum communication systems. It addresses the critical need for high-fidelity entangled photon sources, which are essential for various quantum applications, including entangling sensor networks, quantum computer networks, and quantum key distribution for secure communications.
As quantum technologies continue to evolve, this source array technology positions itself as a crucial component in the development of large-scale, efficient quantum networks, offering a solution to one of the fundamental challenges in quantum information transmission.

Compact, Temperature-Tuned OFDR Laser
Because OFDR-based fiber interrogation systems rely upon interferometry between sensors with respect to a unique reference length, the excitation source (laser) must lase at a single longitudinal mode (SLM). If the excitation source contains multiple modes, the resulting beat frequency becomes a super-position of the multiple frequencies caused by the modes; as a result, the sensor cannot be accurately defined in the Fourier domain. For OFDR systems with high sensing ranges, a continuous wavelength tunable laser must be used to accommodate the resonant wavelength shift of the fiber sensors due to environmental changes. External cavity lasers (ECLs) have been used due to their narrow linewidth and ability to lase at a SLM with no mode-hopping between steps. However, the mechanical complexity associated with tuning, susceptibility to vibration and shock, and high price point leave much to be desired.
To overcome the limitations of OFDR-based FOSS systems resulting from non-ideal excitation sources, NASA has developed a narrow linewidth solid-state laser based on the Distributed Feedback (DFB) laser. NASAs laser is continuously tuned by manipulating the laser cavitys temperature via a thermal-electric cooler feedback system. This continuous wavelength tuning generates a clean clock signal within an auxiliary interferometer, while the laser simultaneously interrogates multiple FBGs to produce a clean sensing interferometer. A Fourier domain spectrograph is used to show the unique frequency (i.e., location) of each FBG.
While NASAs excitation source provides several performance advantages over conventional lasers used in OFDR, it is also highly compact and one eighth the cost of the ECLs traditionally used as excitation sources in OFDR-based systems. The laser has no moving parts, which also substantially improves system reliability.
Originally developed to demonstrate a low-cost interrogator for liquid level sensing in oil tanks, NASAs compact, temperature-tuned OFDR laser can be applied wherever OFDR-based fiber optic sensing is desirable. Additional applications may include temperature distribution sensing, strain sensing, pressure sensing, and more.
NASA AFRC has strong subject matter expertise in fiber optic sensing systems, and has developed several patented technologies that are available for commercial licensing. For more information about the full portfolio of FOSS technologies, visit:
https://technology-afrc.ndc.nasa.gov/featurestory/fiber-optic-sensing

Serial Arrayed Waveguide Grating
Serial Arrayed Waveguide Grating enables higher resolution wavelength separation. Traditional AWGs split the optical signal into multiple parallel paths each with a different path length. This new approach creates the different path lengths by splitting the signal into essentially one long path in which the different channels are periodically split off the main path in the desired fraction. This has the net result of requiring much less space on-chip for comparable optical path differences.
In traditional AWG, there are multiple parallel optical paths, each with a different engineered path-length. For high resolution, you want many different parallel paths and large differences in path length between the paths. To design this on a photonics chip requires significant area. The serial AWG creates a single path, equivalent to the longest path in the parallel AWG and split off fractions of the optical signal at various points along the way to create the equivalent path lengths. Serial Arrayed Waveguide Grating re-uses the same path instead of needing independent parallel paths.

Receiver for Long-distance, Low-backscatter LiDAR
The NASA receiver is specifically designed for use in coherent LiDAR systems that leverage high-energy (i.e., > 1mJ) fiber laser transmitters. Within the receiver, an outgoing laser pulse from the high-energy laser transmitter is precisely manipulated using robust dielectric and coated optics including mirrors, waveplates, a beamsplitter, and a beam expander. These components appropriately condition and direct the high-energy light out of the instrument to the atmosphere for measurement. Lower energy atmospheric backscatter that returns to the system is captured, manipulated, and directed using several of the previously noted high-energy compatible bulk optics. The beam splitter redirects the return signal to mirrors and a waveplate ahead of a mode-matching component that couples the signal to a fiber optic cable that is routed to a 50/50 coupler photodetector. The receiver’s hybrid optic design capitalizes on the advantages of both high-energy bulk optics and fiber optics, resulting in order-of-magnitude enhancement in performance, enhanced functionality, and increased flexibility that make it ideal for long-distance or low-backscatter LiDAR applications.
The related patent is now available to license. Please note that NASA does not manufacturer products itself for commercial sale.