Next Generation “Closed Strayton” Engine Design
Power Generation and Storage
Next Generation “Closed Strayton” Engine Design (LEW-TOPS-168)
Genset Delivers Compact, High-Efficiency Power for the Future
Overview
NASA’s Glenn Research Center introduces the “Closed Strayton” generator design to efficiently deliver lightweight and sustainable electric power for clean energy applications. Optimized for hydrogen-based, zero-emission electrified aircraft propulsion from kW to MW range, the design builds on the core “Strayton” engine technology, which combines both Stirling and Brayton cycle elements to overcome the size and performance limitations of conventional turbines and heat engines.
In its closed-cycle configuration, the design provides fuel-source agnostic, maintenance-free, quiet power generation for applications with challenging footprint and noise constraints. With additional support for open-cycle and combined-cycle implementations, as well as the capability to scale to higher power outputs, this early-stage technology offers broad applicability for both today and tomorrow’s clean energy and power systems.
The Technology
The core “Strayton” generator technology consists of a gas turbine engine with short, axial pistons installed inside the hollow turbine shaft. These pistons form a Stirling engine that cycles via thermo-acoustic waves, transferring heat from the turbine blades to the compressor stage, which improves overall engine performance. Power to an alternator is, thus, delivered from both turbine shaft rotation and the oscillation of the internal pistons.
This synergistic relationship is markedly enhanced in a closed-cycle system, where the sealed turbine engine recirculates a working fluid heated via an external source, such as a hydrogen fuel cell and combustor. This system supports higher compression ratios, reduces the turbine diameter to less than 4”, and eliminates the need for large recuperators. Operational efficiency is projected to extend into the low temperature range (750° C), reducing the need for advanced materials and providing cleaner combustion for hydrogen-based applications. Pressurized, inert working fluids also replace mechanical bearings and gearboxes, enabling years of maintenance-free operation.
The fuel cell and Stirling cycle produce 10% of the total system energy, while the Brayton cycle produces 90%. Other external heat sources could include nuclear, solar, or biogas. Conservative estimates for the hydrogen fuel-cell configuration lifetime are in the 100,000 hour range.
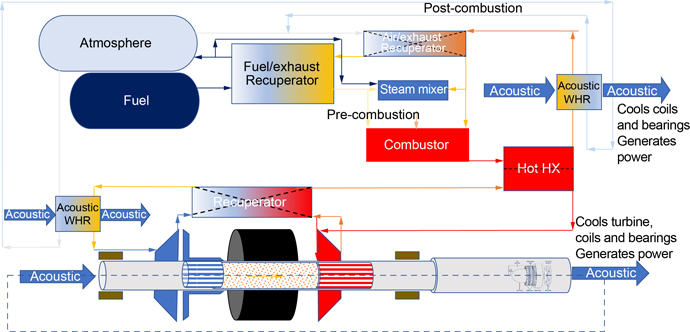
Benefits
- High Efficiency: Over 60% system efficiency with zero-emission option
- Long-Lasting Performance: Sealed system engine uses inert gas bearings for quiet, low maintenance operation
- Compact Power: Shaft-embedded Stirling engine saves weight and size over existing closed-cycle designs
- Versatility: Closed-cycle application supports a wide range of heat sources without the need for advanced alloys
- Low Emissions: Increased performance at lower combustion temperatures reduce NOx emissions
Applications
- Aerospace: Electric Aircraft
- Automotive: Electric Vehicles & Trucks
- Marine: Electric Propulsion & Power
- Generators: C&I On-site Power and Peak Shaving
- Clean Energy: Low-Emission Microturbine Systems
Technology Details
Power Generation and Storage
LEW-TOPS-168
LEW-20416-1
Dyson, R. W. (2023, April 26). True zero emission electric aircraft propulsion transport technology. [Conference Paper] AIAA/IEEE Electric Aircraft Technologies Symposium (EATS) https://ntrs.nasa.gov/citations/20230006407
Dyson, R. (2023, June 14). True zero emission electric aircraft propulsion transport technology [PowerPoint Slides]. American Institute of Aeronautics and Astronautics, Inc. https://ntrs.nasa.gov/citations/20230006821
Tags:
|
Similar Results

High-Voltage Power System for Hybrid Electric Aircraft Propulsion
Glenn's novel system supports the NASA Aeronautics Research Mission Directorate (ARMD) strategic plan to leverage advancements in technologies over the next 25 years and beyond, leading to new aircraft configurations with enhanced performance, improved energy efficiency, and reduced CO2 emissions. The electric system is a multi-megawatt micro-grid that converts mechanical energy to electric via generators, and electric energy to mechanical via motor-driven fans. This innovation would use the variation in aircraft throttle settings to produce a high-voltage (20 kilovolts), variable-frequency 9-phase AC distribution system. Using doubly fed electric machines (generator, propulsor, and flywheel) allows for field excitation that can cause variable-frequency or variable speed operation around the commanded throttle setting. The flywheel enables an energy storage system that recovers and reuses energy, while the flywheel slews with the throttle control using the electromagnetic torque produced by the doubly fed electric machine. This design permits both sub-synchronous and super-synchronous operation using limited field excitation power provided through power converters. Finally, the reduced switchgear mass facilitated through the use of a high-frequency AC system, setting-less protection zones, and simplified switches for fault clearance provides enhanced operational capability. This system can be controlled so that fault energy is minimized, preventing collateral damage to aircraft structures even with high voltage distribution. Glenn's innovative system adds performance, efficiency, reliability, and cost savings to cutting-edge hybrid electric technology.
This is an early-stage technology requiring additional development, and Glenn welcomes co-development opportunities.

High Efficiency Megawatt Motor
The HEMM is a is a wound-field partially superconducting machine that implements a combination of rotor superconducting and stator normal conductor elements, along with an integrated acoustic cryocooler, to achieve some of the benefits of a superconducting motor without the need for an external cryogenic system. The combination of the described elements allows a motor to be built which essentially operates like any other motor when viewed as a black box, but substantially enhanced performance can be achieved. The incorporation of superconductors on the rotor to create a high-level magnetic field results in a specific power and efficiency that could not be achieved any other way. The HEMM can achieve over 98% efficiency in a lightweight electric machine with an operating power greater than 1.4 MW, a specific power greater than 16 kW/kg (ratio to electromagnetic mass), and a rated operating speed of 6800 RPM. The HEMM can be used as both a motor or a generator, offering a wide range of applications including propulsion systems for hybrid aircraft, electric trains, hybrid cars, and turboelectric ships, as well as generator systems for wind turbines, power plants, or motors for other industrial machinery.

Stirling Thermoacoustic Power Converter and Magnetostrictive Alternator
Glenn's thermoacoustic power converter reshapes the conventional Stirling engine from a toroidal shape into a straight colinear arrangement. Instead of relying on failure-prone mechanical inertance and compliance tubes, this design achieves acoustical resonance by using electronic components. In a typical Stirling engine, the acoustical wave travels around a toroid and reflects back, forming a standing wave. In Glenn's device, by contrast, the wave instead travels in a straight plane where a transducer receives the acoustical wave and electrical components modulate the signal. A second transducer on the diametrically opposed side reintroduces the acoustic wave with the correct phasing to achieve amplification and resonance. Glenn's design allows the transducers to operate at high frequency while presenting a mass rather than stiffness impedance.
Glenn's magnetostrictive alternator uses stacked magnetostrictive materials under a biased magnetic and stress-induced compression. The acoustic energy from the engine travels through an impedance-matching layer (which can be formed from aerogel materials) that is physically connected to the magnetostrictive mass. Compression bolts keep the structure under compressive strain, allowing for the micron-scale compression of the magnetostrictive material and eliminating the need for bearings. The alternating compression and expansion of the magnetostrictive material creates an alternating magnetic field that then induces an electric current in a coil wound around the stack. This alternator produces electrical power from the acoustic pressure wave and, when the resonant frequency is tuned to match the engine, can replace the linear alternator to great effect.

Axial Magnetic Flux Airflow Integrated Compressor-Generator-Motor Turbojet
The innovation uses the rotating blades of the compressor section to act as structural support for the generator. Since the compressor is the coolest part of the engine, it will reduce the potential for interference with magnetics and associated curie points of the permanent magnets. The placement of the generator in the cooler part of the engine flowpath (fan or compressor) will also improve the electrical insulation system's degradation and serve to improve overall system lifetime.
The configuration proposed by Armstrong's design would be an axial magnetic flux permanent magnet generator or motor. The electrical/mechanical interface could serve to deliver power to the shaft of the turbojet/fan or extract power from the shaft.
This axial electromagnetic flux design is more efficient for the combined function of aero-thermal heat transfer and generation of electricity. This is due to the relative amount of available cooling surface area, which has an advantage over radial designs given the total system volumetric aspect ratio of the generator/compressor section. When the system is viewed as a thermodynamic cycle, it is more efficient because it is essentially a regenerative cycle, with the heat of generation being fed back into the cycle instead of being released into the ambient surroundings

Thermal Management for Aircraft Propulsion Systems
Aircraft thermal management systems typically comprise over half the mass associated with full electric power propulsion systems, with significant negative impact on fuel efficiency. In addition, the traditional method of using jet fuel to cool aircraft generators does not provide enough cooling for use in flight-weight cryogenic systems. Lastly, the much higher bus voltages required for flight-weight systems (4.5 kV vs. 270 V) introduce additional spark-ignition hazards associated with alternative cryogenic cooling fuels, including liquid methane or liquid hydrogen. The Glenn flight-weight thermal management system addresses all of these problems by using the considerable waste heat energy from turbogenerators to create a pressure wave thermoacoustically. This wave can then be delivered quietly and efficiently via routed ductwork to hollow pulse-tube coolers located near any component in the aircraft that requires cooling. The tubes can be fabricated in any length and can be curved to fit any space. This technology also allows waste heat energy to be used in at least four ways: 1) the waste heat energy can drive a thermoacoustics-based ambient or cryogenic heat pump; 2) it can be channeled directly into a thermoacoustic engine that generates power; 3) it can convectively preheat the fuel/ or air supplied to the aircraft engine; 4) it can drive a pulse-tube generator providing power. The delivered thermoacoustic power can provide cabin cooling as well as ambient/cryogenic cooling of converter, cables, and motors. In addition, this power can be converted to local electric power through the use of a transducer (such as a linear alternator) or piezoelectrics. Further, the efficient thermal management system enables the size, mass, and resultant cost of the radiating fins to be reduced. Glenn's system offers an efficient method of cooling next-generation flight-weight electric aircraft with significant benefits for fuel efficiency and safety.