Luminescence-Based Temperature Mapping and Sensing Systems
sensors
Luminescence-Based Temperature Mapping and Sensing Systems (LEW-TOPS-60)
Luminescence-based temperature mapping using Cr:GdAlO3 is capable of measuring temperatures up to 1300°C
Overview
NASA's Glenn Research Center has developed non-contact, ultra-bright luminescence-based surface temperature mapping and sensing systems capable of operating in environments with extremely high thermal radiation. This is accomplished through the use of a unique chromium-doped gadolinium aluminate (Cr:GdAlO3) temperature-sensing phosphor. This technology has been proven accurate up to 1300°C - a dramatic increase when compared to current state-of-the-art, which has only been demonstrated up to 600°C. In addition to providing breakthrough temperature measurement capability, this innovation is immune to electromagnetic interference, making it ideal for operation in harsh, high-temperature environments. Furthermore, its unprecedented ultra-bright intensity allows for accurate temperature measurements in the presence of high levels of background radiation.
The Technology
In order to obtain real-time temperature measurements with this technology, the phosphor can either be applied as a coating onto the surface of the object for temperature mapping, or incorporated onto a sensor and attached to the end of a fiber-optic probe for local temperature measurements. Next, the object is exposed to a pulsed light source, which causes the temperature-sensing phosphor to produce an ultra-bright broadband emission. An algorithm is used to evaluate post-pulse emission decay time, which is then converted to a precise temperature reading.
State-of-the-art temperature sensing phosphors suffer from thermal quenching, or unacceptable loss of signal intensity as temperature increases. Alternative high-temperature measurement systems such as thermocouples and pyrometers, offer only spot measurements. Moreover, thermocouples suffer from attachment issues and electromagnetic interference, while pyrometers frequently suffer from reflected radiation interference and unknown surface emissivity. Cr:GdAlO3, which retains ultra-bright emission intensity to temperatures well above 1000°C, provides an ideal solution to all of these issues with a few added benefits. It has a perovskite structure that is both non-reactive and stable in high-temperature environments. Furthermore, it possesses a favorable electron energy level spacing that enables this sensor to maintain stronger signal intensity than its competitors. Due to the broad absorption and emission bands for Cr:GdAlO3 there is considerable flexibility in the choice of excitation and emission wavelength detection bands. The combination of these factors makes this technology a clear choice for luminescence-based optical high-temperature sensing for a variety of industries from aerospace to manufacturing.
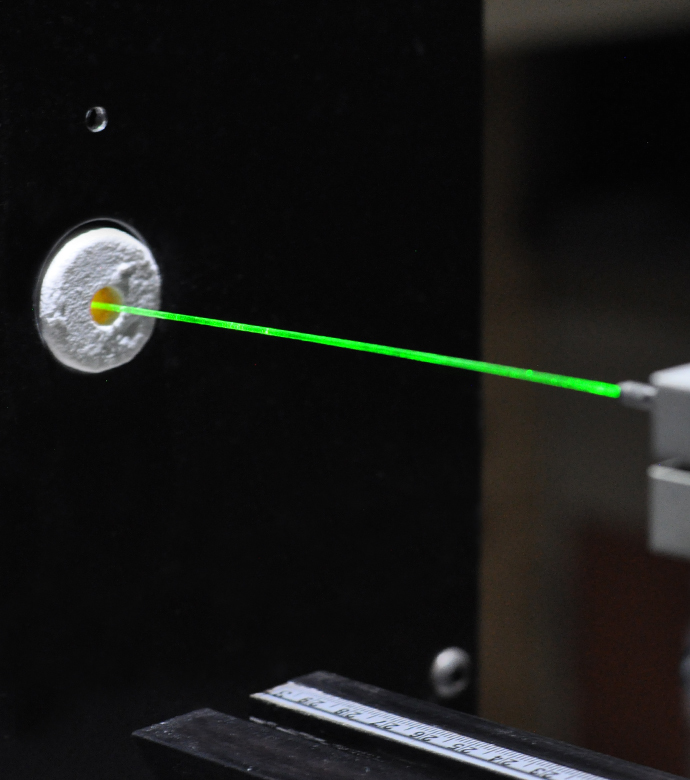
Benefits
- Durable: Measures temperatures up to 1300°C in real- time
- Robust: Ideal for high-temperature, high-radiation, harsh environments
- Reliable: Immune to electromagnetic interference and chemical/thermal degradation
- Versatile: Can be used for full-field surface temperature mapping, as a temperature probe, or as a pressure sensor
Applications
- Oil and gas
- Industrial machinery
- Furnaces
- Turbines
- Power (e.g., generators, nuclear reactors, power plants)
- Chemical and material processing
- Propulsion (i.e., rockets)
- Military (e.g., missiles, ballistics)
- Sensors (i.e., optical thermometers)
Similar Results

Ruthenium-Doped Thermoelectric Materials
Solid state power conversion devices, such as thermoelectrics, depend upon temperature gradients for their operation. For example, aeronautic gas turbine engines maintain the necessary temperature gradients throughout their systems due to the enthalpic processes of combustion, which offers the possibility of generating electrical power for use in primary and secondary electrical systems in the aircraft. However, until now thermoelectric materials have not been able to withstand the combination of high temperatures and oxidative environments present in gas turbine engines. Glenn's innovation overcomes these limitations by using a doped oxide pyrochlore (crystal compound) semiconductor as the thermoelectric material. The material has a low thermal conductivity, which allows it to maintain a thermal gradient and sufficient electrical conductivity to produce an electromotive force. The pyrochlore allows the thermoelectric material to be present within a gas turbine engine, converting heat directly into electricity and functioning at high temperatures without oxidizing in air. Glenn's innovative thermoelectric material permits the benefits of solid-state power conversion devices to improve fuel efficiencies for a broader range of applications than has ever been possible. This innovation is in the early stages of development, and Glenn welcomes opportunities for co-development.

Wireless Temperature Sensor Having No Electrical Connections
This technology is a new sensor made up of dielectric materials tuned to accurately measure a variable and wide range of temperatures. The sensor is wireless and is powered by an external magnetic field. As the temperature changes, the dielectric material changes its signature magnetic response and the change is detected by a magnetic field response sensor. Applications for this technology are temperature sensors for non-conductive surfaces where the conditions or operations require a robust and wireless sensor.

Thin Film Sensor for Ultra High-Temp Measurement
The thin film sensor’s principal advantage lies in its potential to take high frequency temperature measurements from the surface of a reentering spacecraft while simultaneously withstanding the high temperature and oxidizing environment encountered. This data provides engineers with operational phase measurements used to refine the spacecraft’s operational envelope and track flight hardware behavior in addition to providing high frequency temperature measurements that can inform the physics of a boundary layer.
Mismatches in coefficients of thermal expansion (CTE) are expected in TPS-based sensor applications because the metallic materials used for temperature sensing have thermal expansion rates that differ from the rates of the substrate and coating materials in the TPS. At high temperatures during reentry, this mismatch in CTE can create a significant strain differential between the metallic sensor, sensor leads, and the materials to which the sensor and leads are bonded.
High frequency response temperature measurements on the surface of entry spacecraft are not currently possible above ~700 F with existing measurement capabilities. This shortcoming is primarily due to the need for robust sensor behavior at temperatures of several thousand degrees F. The sensor design of this technology preserves the integrity of sensor components while enhancing its high temperature functionality.
The thin film temperature sensor has a technology readiness level (TRL) 5 (Component and/or breadboard validation in relevant environment) and is now available for patent licensing. Please note that NASA does not manufacture products itself for commercial sale.

Robust Sensors Detect Material Ablation and Temperature Changes
Glenn's breakthrough technology introduces batch-fabricated, miniature sensors embedded and distributed over a large surface area of a material or product during the manufacturing process. The sensors can be utilized for test instrumentation or as an integrated in-situ monitoring system. This integrated manufacturing approach preserves the structural and mechanical system integrity by eliminating the antiquated plug-in approach, invasive machining, manual insertion, and gluing processes currently required to implant sensors into a material. The sensor ladder network of resistors and capacitors breaks down as result of the thermo-physical effects caused by temperature, shock, radiation, corrosion, or other reactions, causing a change in the electrical properties. A processor interprets these changes in the electrical properties and generates a high-resolution, large-area surface profile. The profile demonstrates the amount or rate of material deterioration and temperature change, and is used to optimize geometric structural design, develop materials, predict performance, and make decisions. These sensors play an important role as industries work to realize material performance and product design. This type of monitoring is ideal for infrastructures, nuclear enclosures, or any system susceptible to surface deterioration.

Multi-Spectral Imaging Pyrometer
This NASA technology transforms a conventional infrared (IR) imaging system into a multi-wavelength imaging pyrometer using a tunable optical filter. The actively tunable optical filter is based on an exotic phase-change material (PCM) which exhibits a large reversible refractive index shift through an applied energetic stimulus. This change is non-volatile, and no additional energy is required to maintain its state once set. The filter is placed between the scene and the imaging sensor and switched between user selected center-wavelengths to create a series of single-wavelength, monochromatic, two-dimensional images. At the pixel level, the intensity values of these monochromatic images represent the wavelength-dependent, blackbody energy emitted by the object due to its temperature. Ratioing the measured spectral irradiance for each wavelength yields emissivity-independent temperature data at each pixel. The filter’s Center Wavelength (CWL) and Full Width Half Maximum (FWHM), which are related to the quality factor (Q) of the filter, are actively tunable on the order of nanoseconds-microseconds (GHz-MHz). This behavior is electronically controlled and can be operated time-sequentially (on a nanosecond time scale) in the control electronics, a capability not possible with conventional optical filtering technologies.