Compact, Lightweight, CMC-Based Acoustic Liner
Aerospace
Compact, Lightweight, CMC-Based Acoustic Liner (LEW-TOPS-61)
Lightweight solution to reduce subsonic jet engine noise
Overview
In the wake of recent developments that have reduced fan and jet noise contributions to overall jet-engine noise, aircraft designers are turning their attention toward reducing engine core noise. Innovators at NASA's Glenn and Langley Research Centers are teaming to develop a compact, lightweight acoustic liner based on oxide/oxide ceramic matrix composite (CMC) materials. The CMC acoustic liner has variable-depth channels tuned to reduce broad-spectrum noise in subsonic jet engines. The design of those channels has also been focused on structures that minimize the overall liner thickness. Because the technology uses CMCs, rather than heavy metallic materials, it has the potential to reduce overall engine weight. The oxide/oxide CMC materials are able to withstand extremely high temperatures as well. These CMC acoustic liners can be used in many subsonic jet engines - particularly for next-generation aircraft - to reduce engine core noise. Also, because they can withstand high temperatures, core liners constructed with CMC materials can also provide a thermal barrier.
The Technology
NASA researchers are extending an existing oxide/oxide CMC sandwich structure concept that provides mono-tonal noise reduction. That oxide/oxide CMC has a density of about 2.8 g/cc versus the 8.4 g/cc density of a metallic liner made of IN625, thus offering the potential for component weight reduction. The composites have good high-temperature strength and oxidation resistance, allowing them to perform as core liners at temperatures up to 1000°C (1832°F). NASA's innovation uses cells of different lengths or effective lengths within a compact CMC-based liner to achieve broadband noise reduction. NASA has been able to optimize the performance of the proposed acoustic liner by using improved design tools that help reduce noise over a specified frequency range. One such improvement stems from the enhanced understanding of variable-depth liners, including the benefits of alternate channel shapes/designs (curved, bent, etc.). These new designs have opened the door for CMC-based acoustic liners to offer core engine noise reduction in a lighter, more compact package. As a first step toward demonstrating advanced concepts, an oxide/oxide CMC acoustic testing article with different channel lengths was tested. Bulk absorbers could also be used, either in conjunction with or in place of the liners internal chambers, to reduce noise further if desired.
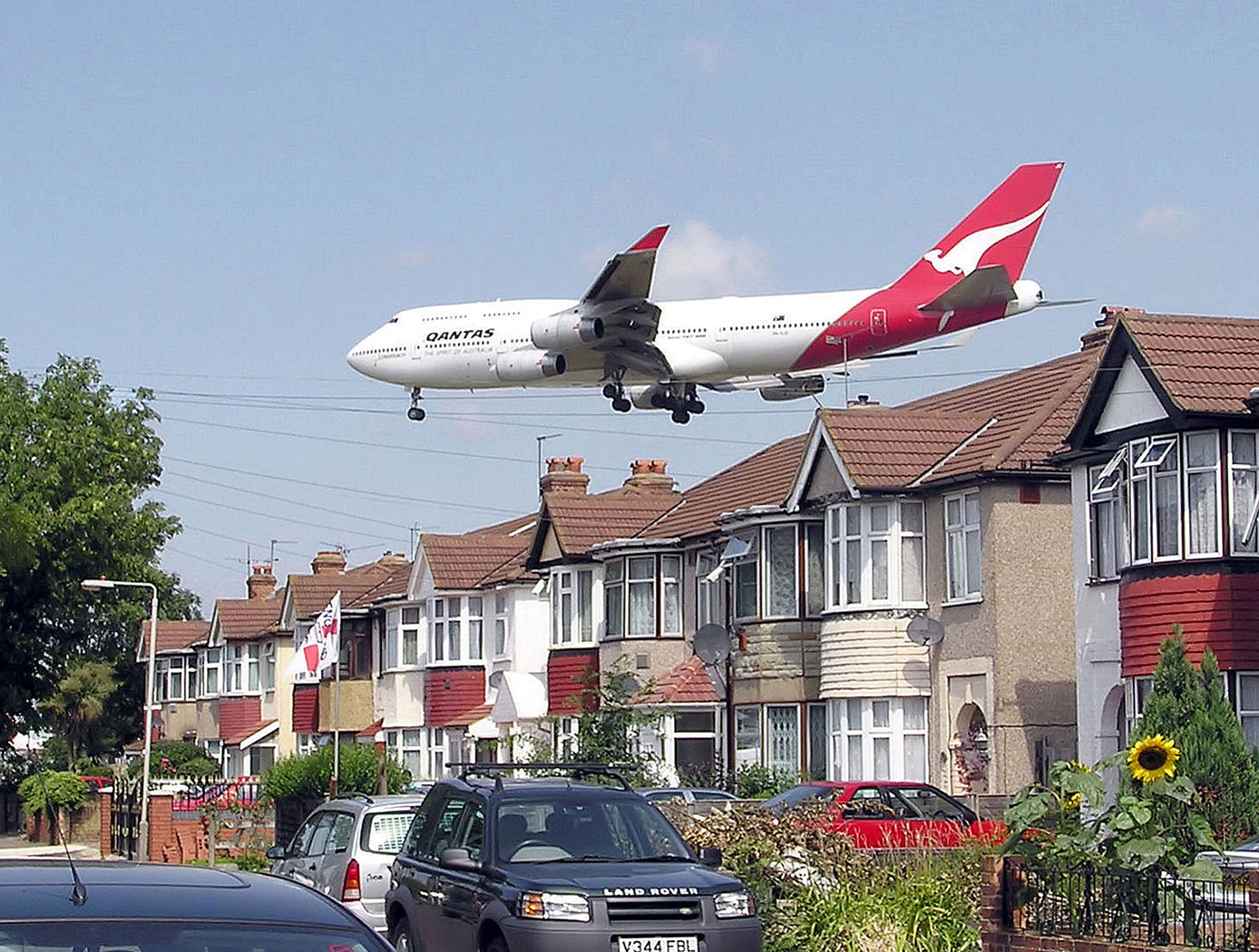
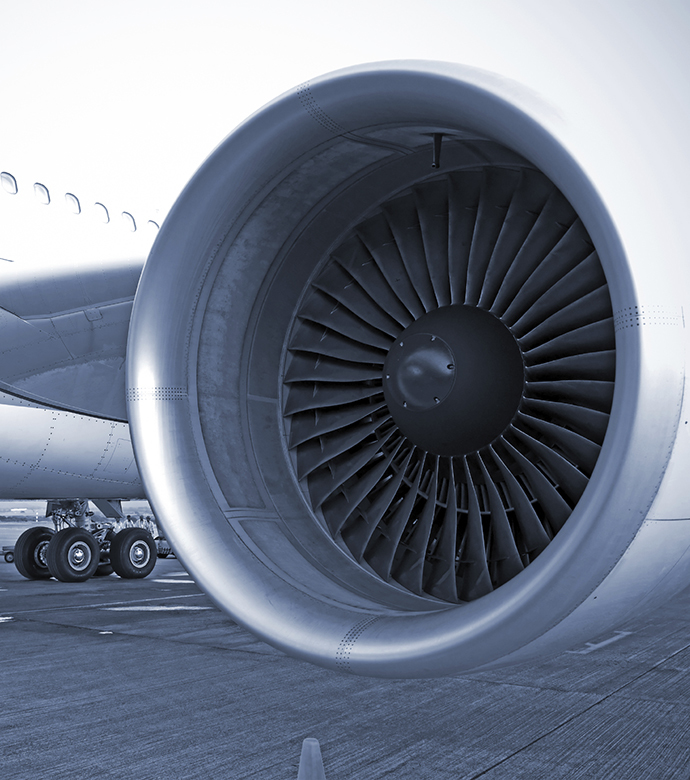
Benefits
- Effective: Reduces noise over a broad frequency range of 2 to 3 octaves
- Lightweight: Uses CMC materials to reduce weight
- Robust: Offers high-temperature capability
Applications
- Subsonic jet engines
Similar Results

Low, Drag, Variable-Depth Acoustic Liner
The drag penalty incurred by a conventional acoustic liner is dependent, to a large extent, on the perforate open area ratio (porosity) of the perforated facesheet. As the open area ratio is decreased, the facesheet behaves more like a solid surface and the drag is reduced. However, if the open area ratio is too small, the external acoustic field will be isolated from the resonators (in the liner), and the system will not provide noise reduction.
The technology is a new type of variable-depth acoustic engine liner, which will reduce the drag and potentially manufacturing cost of this class of engine liner. Individual resonators within a conventional variable-depth liner are effective near resonance, but provide less acoustic benefit at other frequencies. In fact, at anti-resonance, a resonator behaves similar to a hard wall (i.e., the normal component of the particle velocity at the inlet is zero). Therefore, the proposed innovation couples neighboring resonators (tuned for different frequencies) together within the core of the liner. In other words, multiple resonators share a single inlet/port. Sharing inlets reduces the overall number of openings needed to maintain the acoustic performance of the liner by a factor of two or more. Reducing the open area ratio will in turn reduce the liner drag, and will reduce the number of holes that have to be machined into the facesheet, potentially reducing manufacturing cost.
The functional operation of the proposed innovation will be identical to conventional engine liners. The innovation enables a reduction of the open area ratio of the perforated facesheet (by a factor of two or more) without degrading the acoustic performance. This will decrease the liner drag, and has the potential to reduce the manufacturing cost of the liner, since fewer holes need to be machined in the facesheet.

A Method for Reducing Broadband Noise
This NASA technology is ideally suited to absorb sounds below 1000 Hz (at the low end of human auditory range), which commercially available materials have struggled to absorb effectively. NASA innovators designed the acoustic liner to mimic the geometry and the low-frequency acoustic absorption of natural reeds. To provide excellent noise absorption that endures even in a variety of challenging conditions, researchers have created and tested prototypes of acoustic filters using thin and lightweight parallel-stacked tubes one-fourth to three-eights of an inch in diameter. The assembly can feature a porous or perforated face sheet positioned on one or more sides of the acoustic absorber layer to increase noise-reduction capability as needed. These filters have demonstrated exceptional acoustic absorption coefficients in the frequency range of 400 to 3000 Hz. Results indicate that these assemblies can be additively manufactured from synthetic materials, generally plastic; however, ceramics, metals, or other materials can also be used. The reeds can be narrow or wide, hollow or solid, straight or bent, etc., giving this acoustic liner remarkable flexibility and versatility to meet the needs of virtually any application. This technology effectively demonstrates that a new class of structures can now be considered for a wide range of environments and applications that need durable, lightweight, broadband acoustic absorption that is effective at various frequencies, particularly between 400 and 3000 Hz.

Environmental Barrier Coatings for Ceramic Matrix Composites
CMCs are a game-changer for a number of applications because of their lighter weight, higher temperature capability, and resistance to oxidation. It has been estimated that aircraft designs relying on CMCs can decrease fuel consumption by 10% by 2020. EBCs are used to protect CMCs from water vapor and other corrosive gases inside engines and other extreme environments. The current state of the art for EBCS features a silicon bond coat that is not viable beyond its melting point of 1482°C. By contrast, Glenn's EBCs have demonstrated a steam oxidation life of at least 500 hours at 1482°C, making them ideal durable coatings for next-generation CMCs. These EBCs are slurries, with either a mullite-based bond coat or a rare earth disilicate-based bond coat comprising at least three and two layers, respectively. Mullite is often used as a refractory material for furnaces, reactors, etc. because of its high melting point (1840°C). Rare earth disilicates also have high melting points (~1800°C). These bond coats can be fabricated by preparing a mixture of a coating material, a primary sintering aid, at least one secondary sintering aid, and a solvent. The mixture is then processed (e.g., in a milling media) to form a slurry that can be deposited to a CMC substrate. The sintering aids have two primary functions: 1) densifying deposited slurry by generating liquid phases via reactions with the coating material and other sintering aids, so that the liquid fills gaps between particles of coated material; 2) enhancing bonding and performance of the coating by generating reaction products that enhance those qualities. One great advantage of this EBC is that it can be fabricated via various low-cost methods - including dipping, spinning, spin-dipping, painting, and spraying - in addition to plasma-spraying. Glenn's innovation rises to meet the need for a new class of EBCs that can keep up with CMCs' increasing ability to withstand higher temperatures and stresses than ever before.

Silicon Carbide (SiC) Fiber-Reinforced SiC Matrix Composites
Aimed at structural applications up to 2700°F, NASA's patented technologies start with two types of high-strength SiC fibers that significantly enhance the thermo-structural performance of the commercially available boron-doped and sintered small-diameter “Sylramic” SiC fiber. These enhancement processes can be done on single fibers, multi-fiber tows, or component-shaped architectural preforms without any loss in fiber strength. The processes not only enhance every fiber in the preforms and relieve their weaving stresses, but also allow the preforms to be made into more shapes. Environmental resistance is also enhanced during processing by the production of a protective in-situ grown boron-nitride (iBN) coating on the fibers. Thus the two types of converted fibers are called “Sylramic-iBN” and “Super Sylramic-iBN”.
For high CMC toughness, two separate chemical vapor infiltration (CVI) steps are used, one to apply a boron nitride coating on the fibers of the preform and the other to form the SiC-based matrix. The preforms are then heat treated not only to densify and shrink the CVI BN coating away from the SiC matrix (outside debonding), but also to increase its creep resistance, temperature capability, and thermal conductivity.
One crucial advantage in this suite of technologies lies in its unprecedented customizability. The SiC/SiC CMC can be tailored to specific conditions by down-selecting the optimum fiber, fiber coating, fiber architecture, and matrix materials and processes. In any formulation, though, the NASA-processed SiC fibers display high tensile strength and the best creep-rupture resistance of any commercial SiC fiber, with strength retention to over 2700°F.

Autonomous Slat-Cove Filler Device
NASA Langley designed the shape memory alloy slat-cove filler to provide significant broad-band noise reduction to any aircraft wing structure that has a leading-edge, high-lift device and that is distinct from the main-wing element. The design can be retrofitted to existing aircraft structures and can be easily incorporated into the existing or future designs for aircraft wing structures. The concept involves very few components, requires no additional mechanical support from pneumatic or hydraulic systems, and makes use of existing slat-actuation systems for retraction. The design is autonomous, simple, and constitutes low-weight addition. The concept is also considered fail-safe because the lift would not be diminished in the event that the slat cove filler failed to deploy.
Several advancements have been devised to accommodate complex features encountered in application to practical airframe structures. Graphics from a computational model of a 2D physical demonstration system show the configuration and strain in the slat-cove filler in the deployed and stowed conditions. Features enabling stowage of a large curvilinear length (sliding hinge) and maintenance of the optimized outer mold line (auxiliary component) are highlighted. Other advancements for application to 3D, flight airframes are visible in the image from a model for one entire section of a slat-cove-filler treatment for a wide-body, transport-class aircraft.
NASA Langley also offers a design for a deformable structure that is deployed from the leading edge of the main-wing element, termed the slat-gap filler. It closes and covers the gap between the slat and the main-wing element, but can be readily and autonomously opened in emergency to regain the baseline high-lift configuration and its corresponding lift performance at high angles of attack. This approach has similar benefits as the slat-cove filler device.