Search
information technology and software

Meta Monitoring System (MMS)
Meta Monitoring System (MMS) was developed as an add-on to NASA Ames patented Inductive Monitoring System (IMS), which estimates deviation from normal system operations. MMS helps to interpret deviation scores and determine whether anomalous behavior is transient or systemic. MMS has two phases: a model-building training phase, and a monitoring phase. MMS not only uses deviation scores from nominal data for training but can also make limited use of results from anomalous data. The invention builds two models: one of nominal deviation scores and one of anomalous deviation scores, each consisting of a probability distribution of deviation scores. After the models are built, incoming deviation scores from IMS (or a different monitoring system that produces deviation scores) are passed to the learned model, and probabilities of producing the observed deviation scores are calculated for both models. In this fashion, users of MMS can interpret deviation scores from the monitoring system more effectively, reducing false positives and negatives in anomaly detection.
Note: Patent license only; no developed software available for licensing
Manufacturing
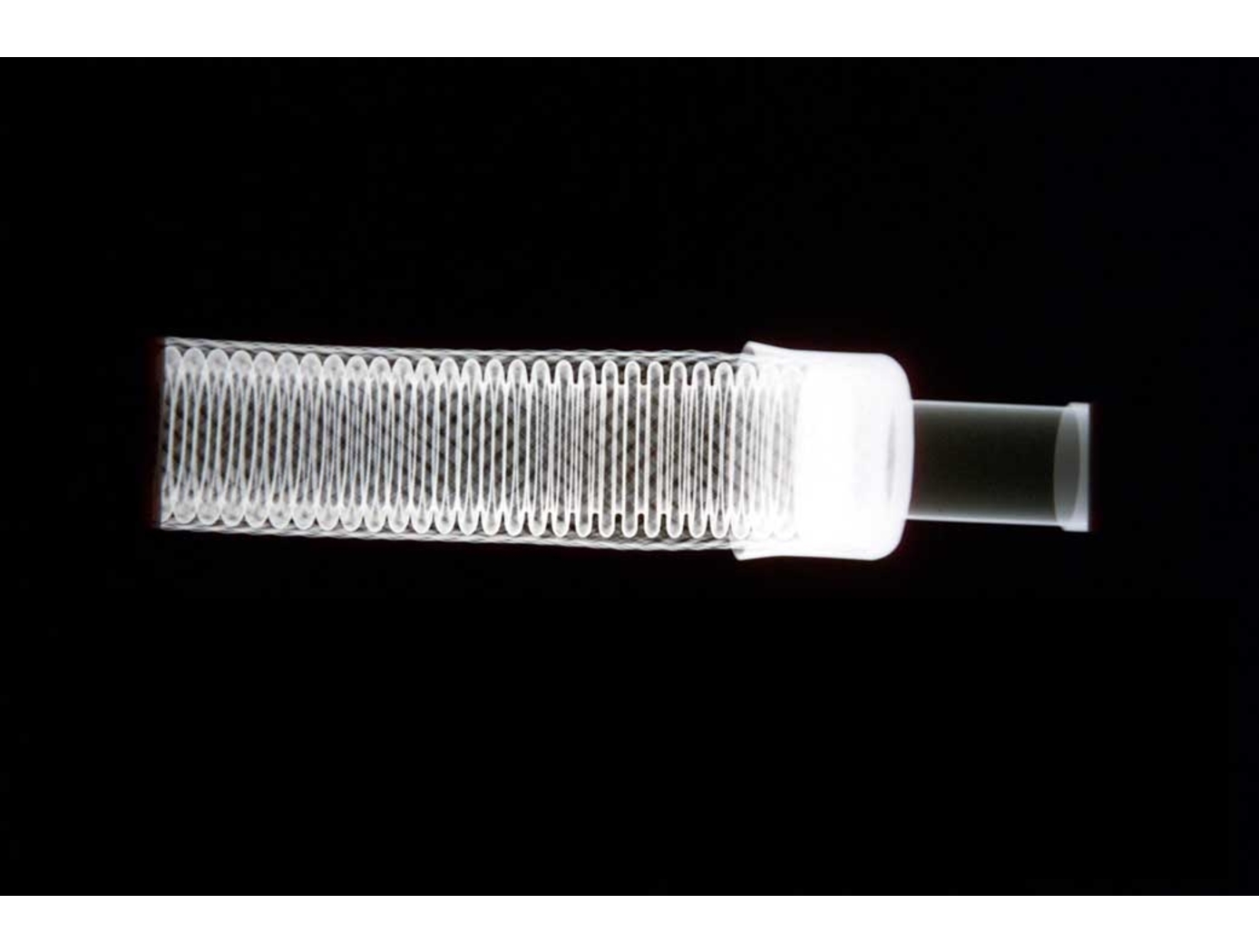
X-Ray Crack Detectability
NASAs software technology uses an Image Quality Indicator (IQI)-based model that can predict whether cracks of a certain size can be detected, as well as a model that can provide appropriate conditions to optimize x-ray crack detection setup. Because this modeling software can predict minimum crack sizes that can be detected by a particular X-ray radiography testing setup, users can test various setups until the desired crack detection capabilities are achieved (predicted) by the modeling system.
These flaw size parameter models use a set of measured inputs, including thickness sensitivity, detector modulation transfer function, detector signal response function, and other setup geometry parameters, to predict the minimum crack sizes detectable by the testing setup and X-ray angle limits for detecting such flaws.
Current X-ray methods provide adequate control for detection of volumetric flaws but do not provide a high probability of detection (POD), and crack detection sensitivity cannot be verified for reliable detection. This results in reduced confidence in terms of crack detection. Given that these cracks, if undetected, can cause catastrophic failure in various systems (e.g., pressure vessels, etc.), verifying that X-ray radiography systems used for NDE can detect such cracks is of the utmost importance in many applications.
Aerospace
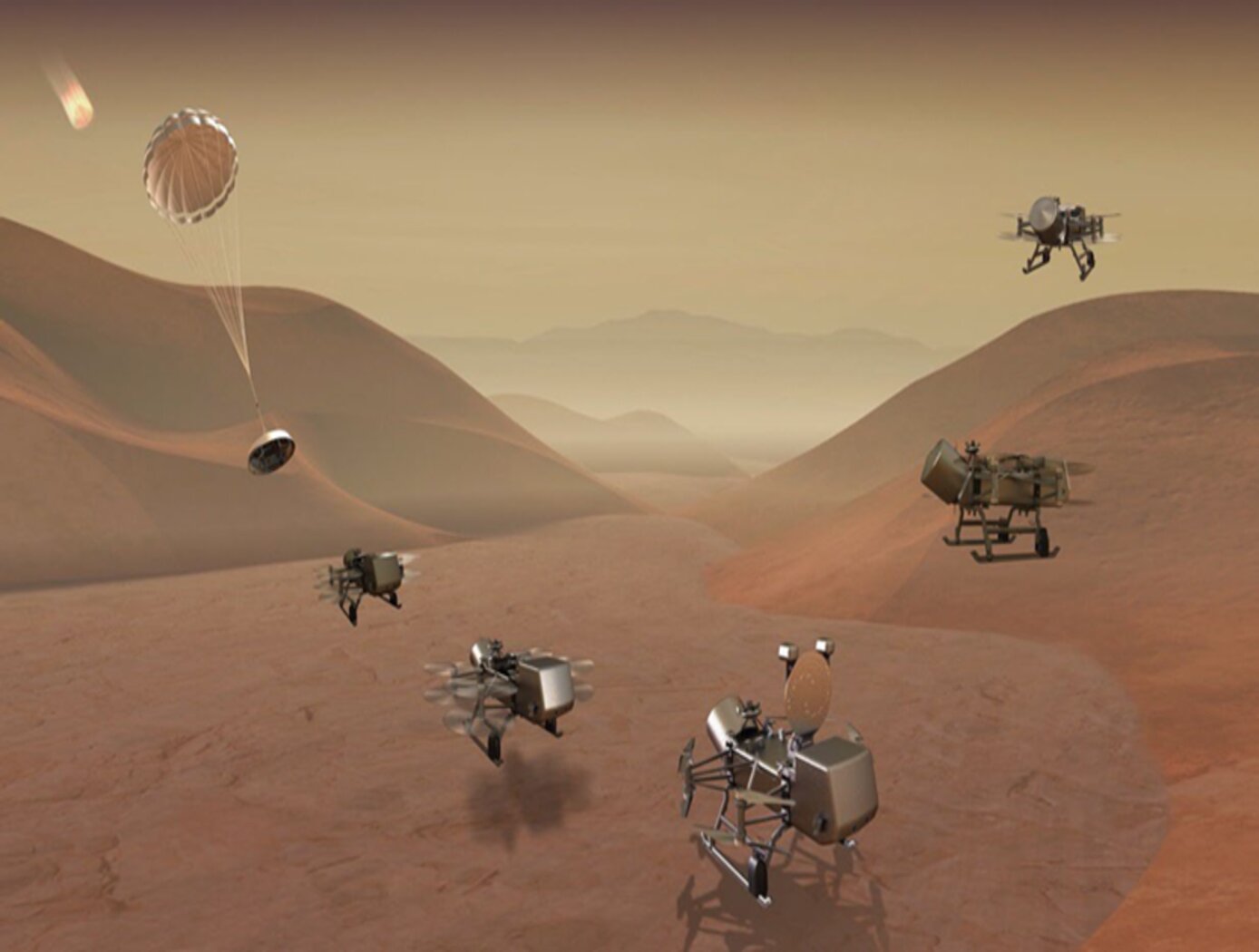
3D Lidar for Autonomous Landing Site Selection
Aerial planetary exploration spacecraft require lightweight, compact, and low power sensing systems to enable successful landing operations. The Ocellus 3D lidar meets those criteria as well as being able to withstand harsh planetary environments. Further, the new tool is based on space-qualified components and lidar technology previously developed at NASA Goddard (i.e., the Kodiak 3D lidar) as shown in the figure below.
The Ocellus 3D lidar quickly scans a near infrared laser across a planetary surface, receives that signal, and translates it into a 3D point cloud. Using a laser source, fast scanning MEMS (micro-electromechanical system)-based mirrors, and NASA-developed processing electronics, the 3D point clouds are created and converted into elevations and images onboard the craft. At ~2 km altitudes, Ocellus acts as an altimeter and at altitudes below 200 m the tool produces images and terrain maps. The produced high resolution (centimeter-scale) elevations are used by the spacecraft to assess safe landing sites.
The Ocellus 3D lidar is applicable to planetary and lunar exploration by unmanned or crewed aerial vehicles and may be adapted for assisting in-space servicing, assembly, and manufacturing operations. Beyond exploratory space missions, the new compact 3D lidar may be used for aerial navigation in the defense or commercial space sectors. The Ocellus 3D lidar is available for patent licensing.
sensors
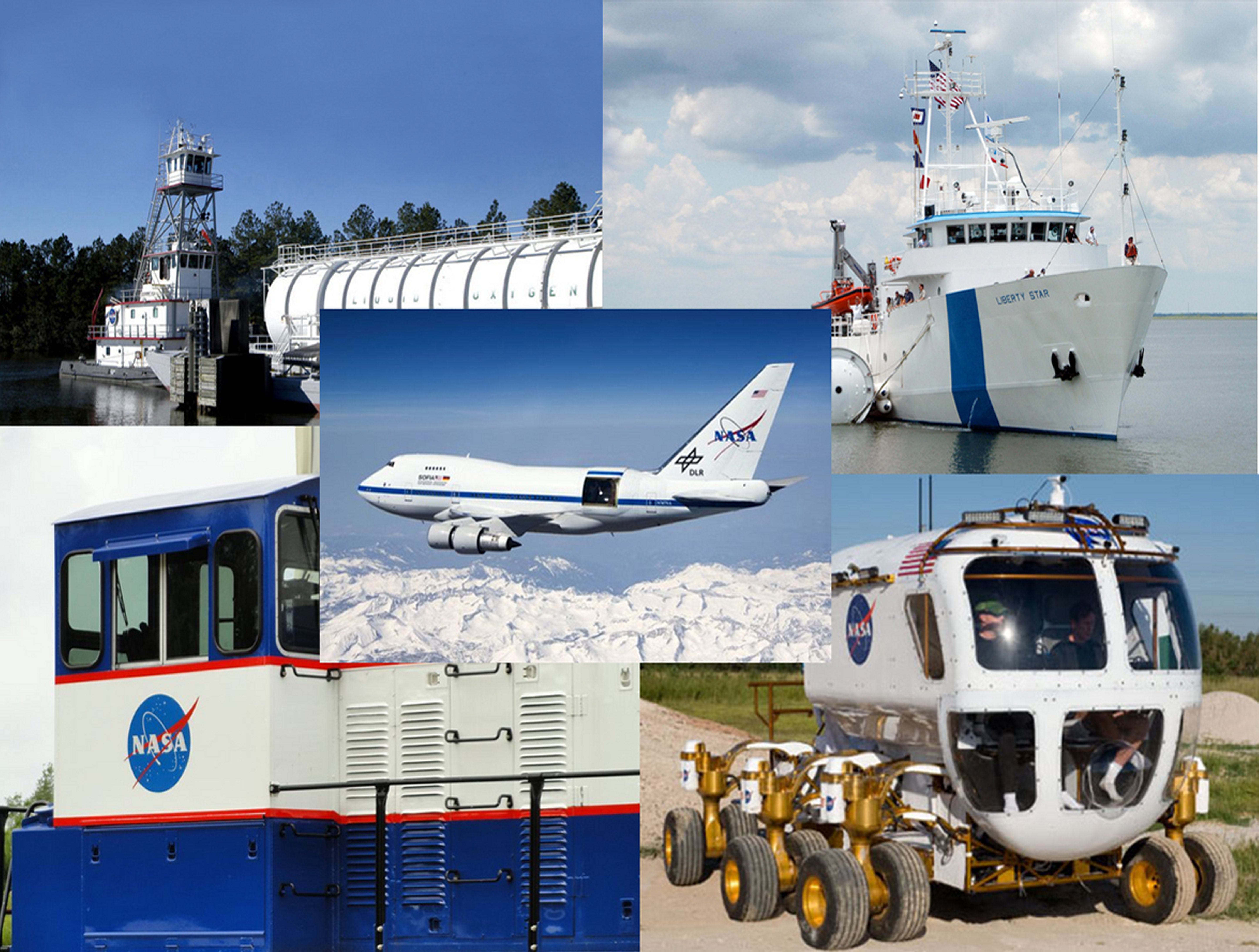
In Situ Performance Monitoring of Piezoelectric Sensors and Accelerometers
On occasion, anomalies may appear in the highly dynamic test data obtained during rocket engine tests, which are investigated and corrective action may be mandated before subsequent testing. Also, it is often unclear if anomalies in recorded signals are due to differences between the Low and High Speed Data Acquisitions Systems, difference between the transducers, a failed transducer, or if everything is working correctly and the system were actually accurately recording real events. Commercial test equipment suitable for testing piezoelectric sensors is expensive and requires that the sensor be removed from the test article for evaluation. With the monitoring system developed, degraded sensor performance can be quickly and economically identified.
This system can evaluate installed piezoelectric sensors, without requiring physical contact with or removing them from their mounted locations. Tests are conducted through cabling. Since it is not necessary to remove the device, data that reflect the devices specific physical configuration (such as as-mounted resonant frequency) are retained, and devices that are physically inaccessible can still be tested. The testing system is not limited to identifying degraded performance in the sensors piezoelectric elements; it can detect changes within the entire sensor, and sensor housing.
The system can be made portable, in a battery powered sealed box, for testing in the field. Since physical contact with the sensor is not necessary, therefore, monitoring can be done as far away as 250 feet, or longer if certain provisions are made.
Optics
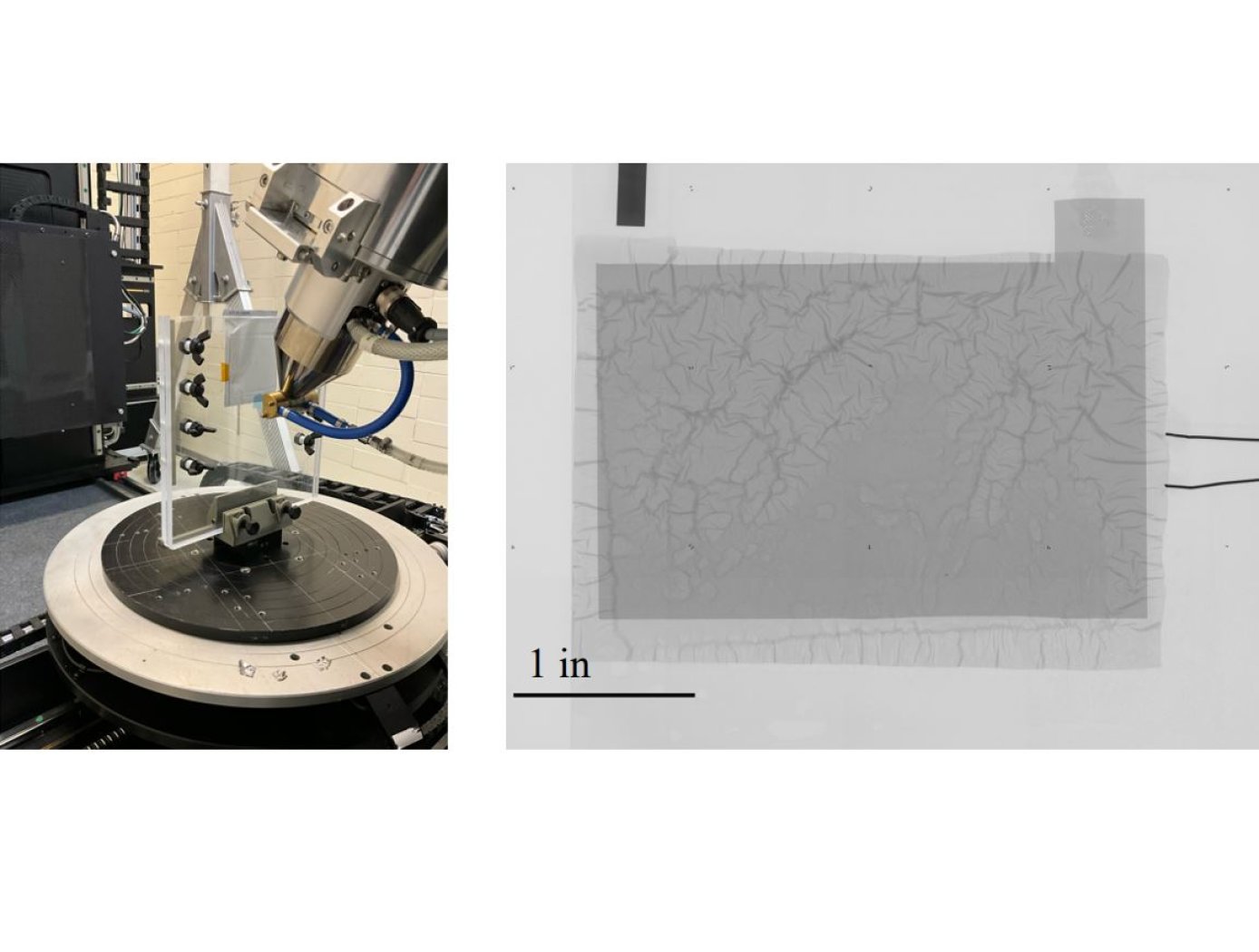
Fingerprinting for Rapid Battery Inspection
The technology utilizes photopolymer droplets (invisible to the digital radiograph) with embedded radiopaque fragments to create randomized fingerprints on battery samples. The droplets are deposited using a jig (see figure on right) that precisely positions samples. Then, at different points during battery R&D testing or use, digital radiography imaging with micron-level resolution can be performed.
The high-resolution imaging required to detect dendrite formation requires images to be collected in multiple “tiles” as shown below. The randomized fingerprints uniquely identify relative positioning of these tiles, allowing rapid assembly of composite high-resolution images from multiple tiles.
This same composite creation process can be used for images taken at a series of points in time during testing, and background subtraction can be applied to efficiently compare how the battery is changing over successive charge/discharge cycles to identify dendrite formation. This inspection technique is proven effective for thin-film pouch cell prototypes at NASA, and it works well at the lowest available x-ray energy level (limiting impact on the samples). The Fingerprinting for Rapid Battery Inspection technology is available for patent licensing.
Optics
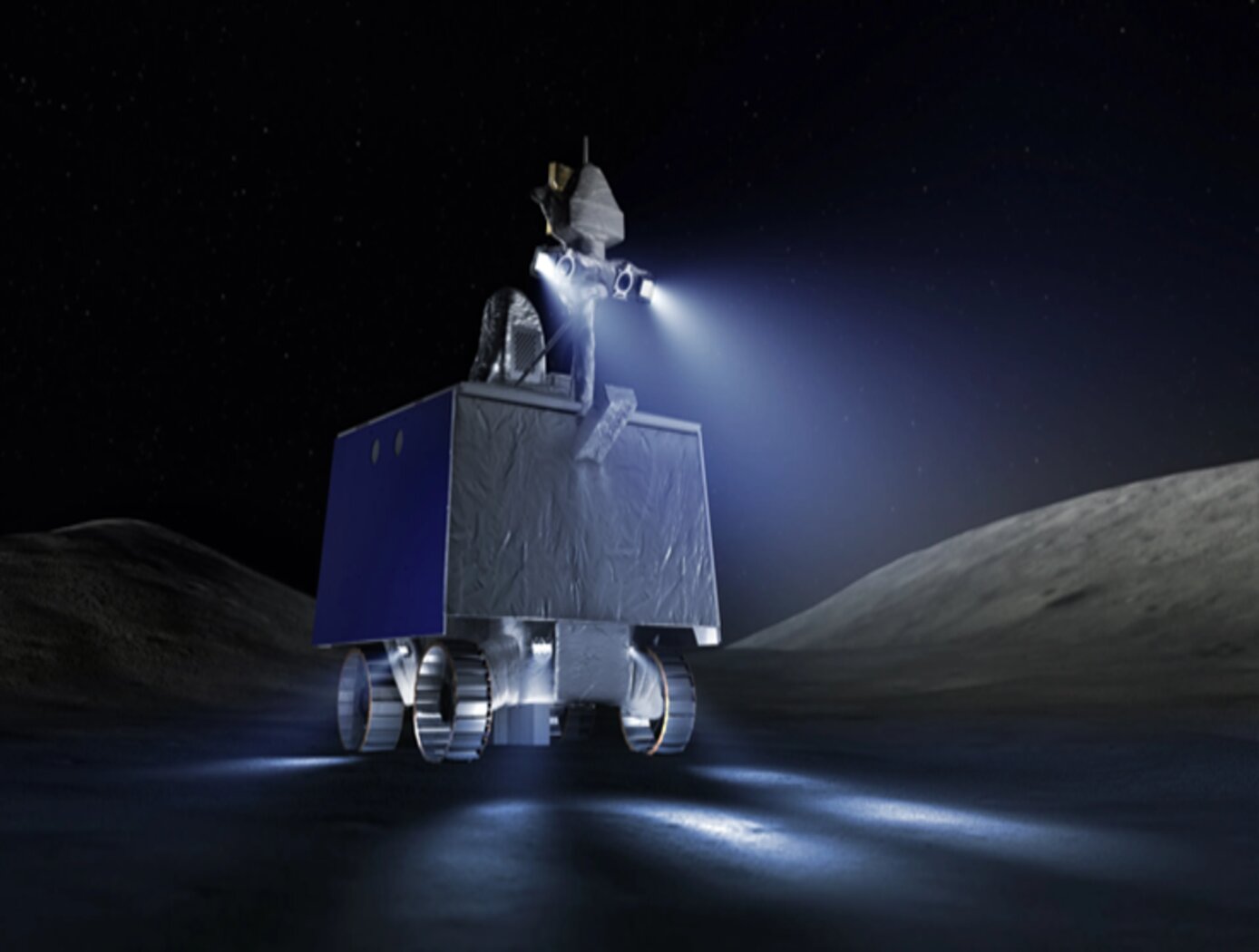
3D Lidar for Improved Rover Traversal and Imagery
The SQRLi system is made up of three major components including the laser assembly, the mirror assembly, and the electronics and data processing equipment (electronics assembly) as shown in the figure below. The three main systems work together to send and receive the lidar signal then translate it into a 3D image for navigation and imaging purposes.
The rover sensing instrument makes use of a unique fiber optic laser assembly with high, adjustable output that increases the dynamic range (i.e., contrast) of the lidar system. The commercially available mirror setup used in the SQRLi is small, reliable, and has a wide aperture that improves the field-of-view of the lidar while maintaining a small instrument footprint. Lastly, the data processing is done by an in-house designed processor capable of translating the light signal into a high-resolution (sub-millimeter) 3D map. These components of the SQRLi enable successful hazard detection and navigation in visibility-impaired environments.
The SQRLi is applicable to planetary and lunar exploration by unmanned or crewed vehicles and may be adapted for in-space servicing, assembly, and manufacturing purposes. Beyond NASA missions, the new 3D lidar may be used for vehicular navigation in the automotive, defense, or commercial space sectors. The SQRLi is available for patent licensing.
manufacturing
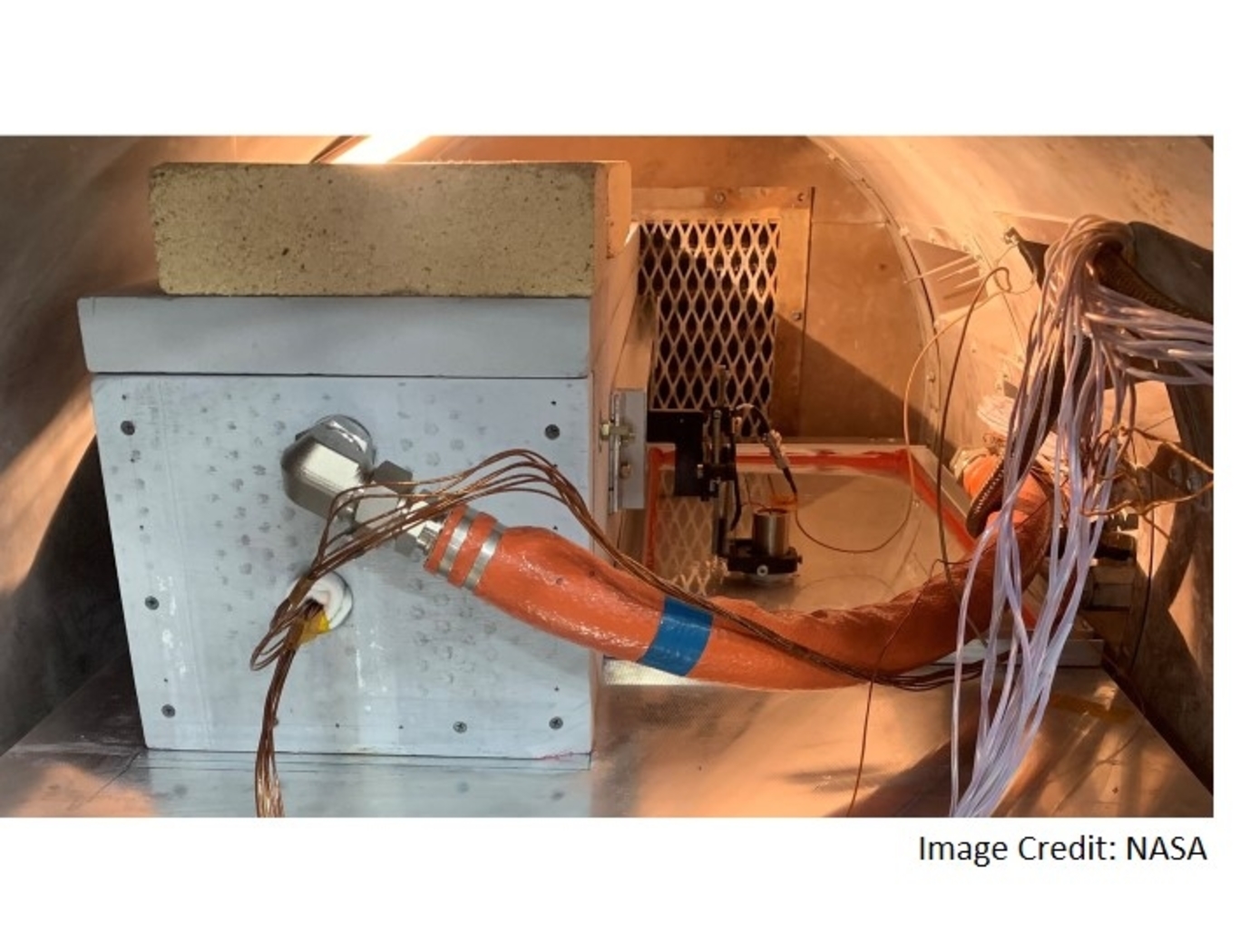
System for In-situ Defect Detection in Composites During Cure
NASA's System for In-situ Defect (e.g., porosity, fiber waviness) Detection in Composites During Cure consists of an ultrasonic portable automated C-Scan system with an attached ultrasonic contact probe. This scanner is placed inside of an insulated vessel that protects the temperature-sensitive components of the scanner. A liquid nitrogen cooling systems keeps the interior of the vessel below 38°C. A motorized X-Y raster scanner is mounted inside an unsealed cooling container made of porous insulation boards with a cantilever scanning arm protruding out of the cooling container through a slot. The cooling container that houses the X-Y raster scanner is periodically cooled using a liquid nitrogen (LN2) delivery system. Flexible bellows in the slot opening of the box minimize heat transfer between the box and the external autoclave environment. The box and scanning arm are located on a precision cast tool plate. A thin layer of ultrasonic couplant is placed between the transducer and the tool plate. The composite parts are vacuum bagged on the other side of the tool plate and inspected. The scanning system inside of the vessel is connected to the controller outside of the autoclave. The system can provide A-scan, B-scan, and C-scan images of the composite panel at multiple times during the cure process.
The in-situ system provides higher resolution data to find, characterize, and track defects during cure better than other cure monitoring techniques. In addition, this system also shows the through-thickness location of any composite manufacturing defects during cure with real-time localization and tracking. This has been demonstrated for both intentionally introduced porosity (i.e., trapped during layup) as well processing induced porosity (e.g., resulting from uneven pressure distribution on a part). The technology can be used as a non-destructive evaluation system when making composite parts in in an oven or an autoclave, including thermosets, thermoplastics, composite laminates, high-temperature resins, and ceramics.
Health Medicine and Biotechnology
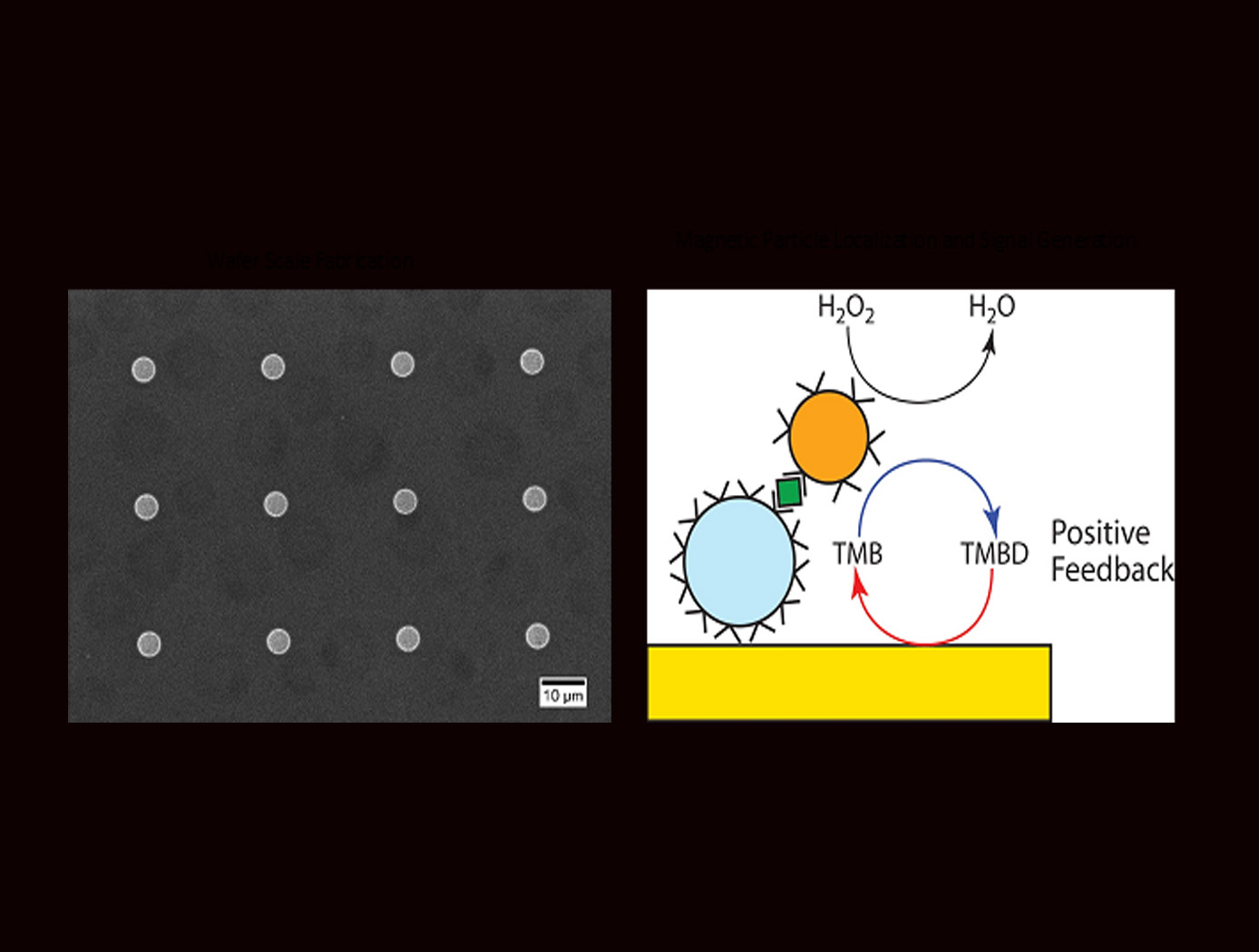
Electrochemical Sensors Based on Enzyme-Linked Immunosorbent Assay
NASA’s electrochemical Enzyme-Linked Immunosorbent Assay (ELISA) microelectrode array biosensor advantageously incorporates a microbead detection construct, coupled with a magnetic immobilization construct, which substantially increases the signal sensitivity of a sensor. The magnetic immobilization construct draws the microbead detection construct to an electrode detection surface, enhancing signal sensitivity. By concentrating the signaling molecules close to the electrode detection surface, electrochemical redox cycling is achieved by reducing the distance between the two, allowing for regeneration of reporter molecules.
Whereas a traditional ELISA testing exhibits five to ten signaling molecules per probe molecule binding event, the present electrochemical ELISA-based biosensor testing exhibits up to 4,857 signaling molecules per probe molecule binding event. The model bead construct exhibits a more than 6.75-fold in increased measured signal, and more than 35.7-fold improvement in signal sensitivity. When compared to traditional optical ELISA, the present invention improves the limit of detection by up to a factor of 60.5.
NASA’s electromagnetic ELISA-based biosensor can be used for the detection of SARS-CoV-2 virus to enhance Covid-19 testing during the early phases of infection. The technology may also be modified to detect other biomarkers.
information technology and software
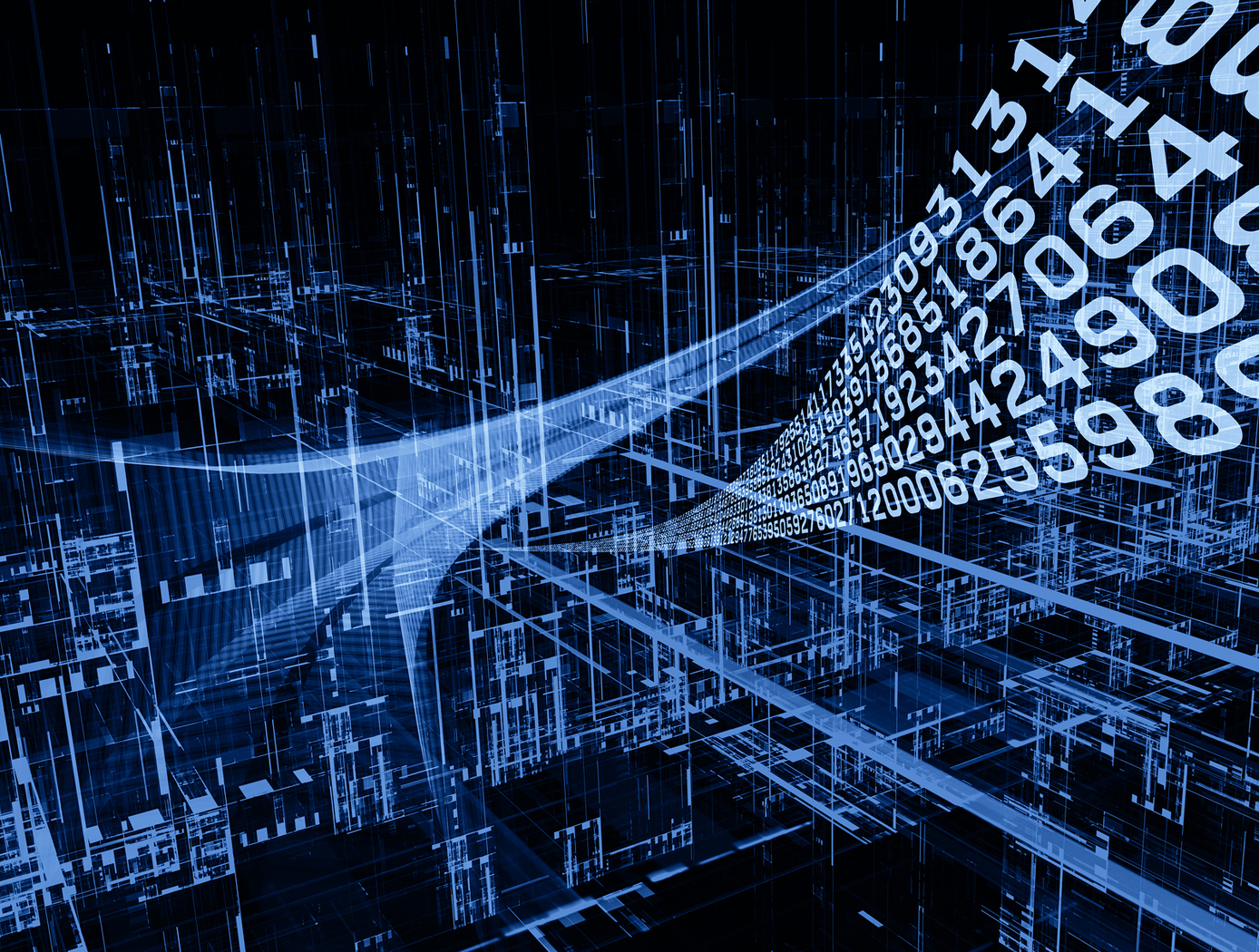
Enhancing Fault Isolation and Detection for Electric Powertrains of UAVs
The tool developed through this work merges information from the electric propulsion system design phase with diagnostic tools. Information from the failure mode and effect analysis (FMEA) from the system design phase is embedded within a Bayesian network (BN). Each node in the network can represent either a fault, failure mode, root cause or effect, and the causal relationships between different elements are described through the connecting edges.
This novel approach can help Fault Detection and Isolation (FDI), producing a framework capable of isolating the cause of sub-system level fault and degradation.
This system:
Identifies and quantifies the effects of the identified hazards, the severity and probability of their effects, their root cause, and the likelihood of each cause;
Uses a Bayesian framework for fault detection and isolation (FDI);
Based on the FDI output, estimates health of the faulty component and predicts the remaining useful life (RUL) by also performing uncertainty quantification (UQ);
Identifies potential electric powertrain hazards and performs a functional hazard analysis (FHA) for unmanned aerial vehicles (UAVs)/Urban Air Mobility (UAM) vehicles.
Despite being developed for and demonstrated with an application to an electric UAV, the methodology is generalized and can be implemented in other domains, ranging from manufacturing facilities to various autonomous vehicles.
Sensors

Hybrid carbon nanotube-gold nanoparticle composite for Nitric Oxide (NO) detection
A hybrid thin film is fabricated by a simple drop-casting method. Functionalized single-walled carbon nanotubes (SWCNTs) and gold nanoparticles (AuNPs) with a diameter of ≈15 nm are drop-casted onto a printed circuit board (PCB) substrate equipped with interdigitated electrodes. The addition of AuNPs to the carbon nanotube networked films enhance sensitivity and lower the detection limit to low parts-per-billion (ppb) concentrations. The gold particle to carbon nanotube ratio is optimized to find the optimum gold nanoparticle loading.
The composite films were tested in both air and nitrogen environments across a wide relative humidity range (0-97%), which is suitable for dissolved Nitric Oxide (NO) detection in sea water for oceanographic study and for human breath analysis in medical diagnosis. The sensors exhibited high selectivity, particularly to NO, outperforming other tested gases. Notably, the sensor reliably detected NO at 10 ppb levels with response times within 10 seconds and recovery time around 1 minute, showcasing excellent reproducibility across sensors and operational efficiency within diverse humidity conditions.