Search
materials and coatings
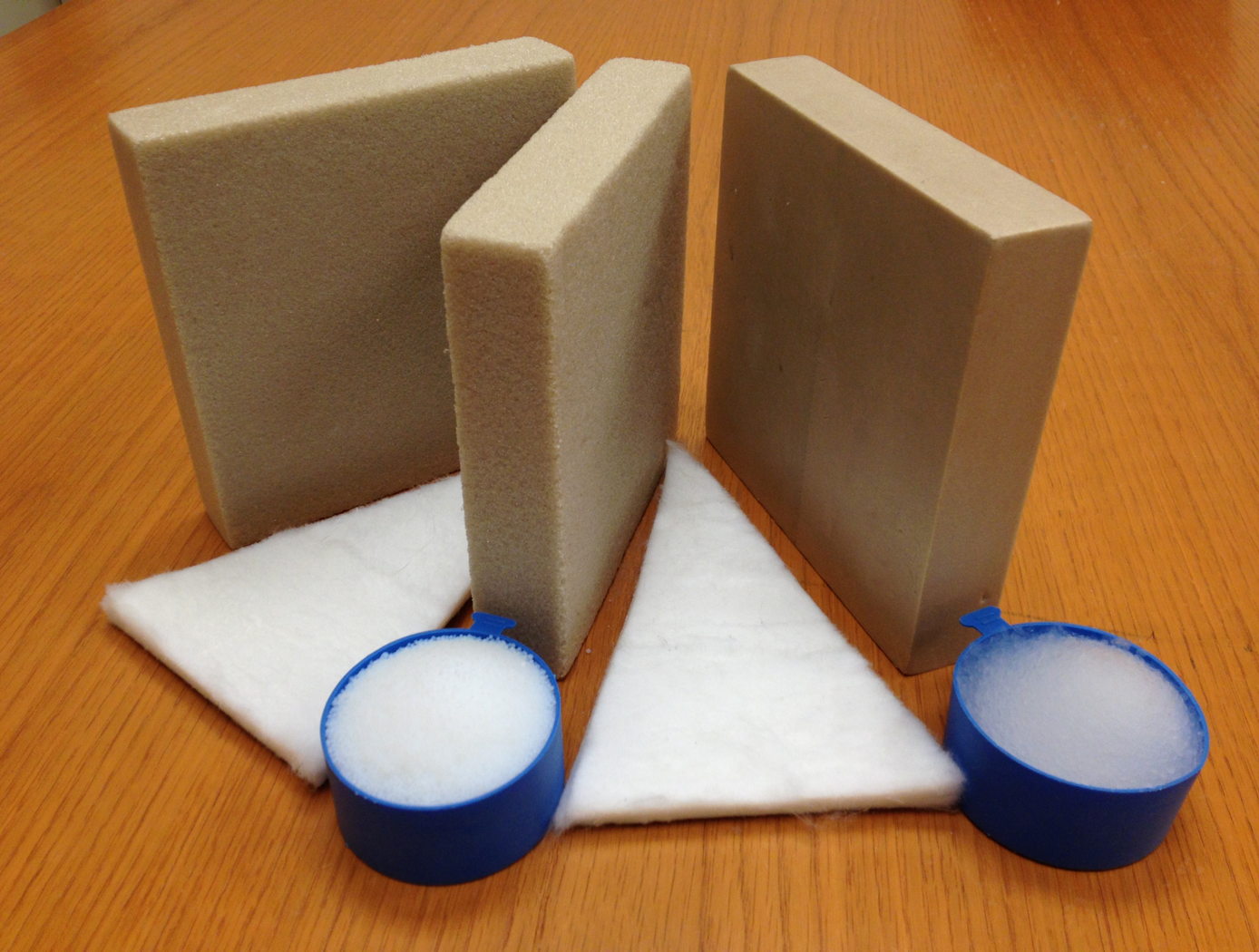
Aerofoam
The Aerofoam composites have superior thermal and acoustic insulation properties when compared to conventional polyimide foams. In addition, they provide greater structural integrity than the fragile aerogel materials can provide independently. In general, polymer foams can provide excellent thermal insulation, and polyimide foams have the additional advantage of excellent high-temperature behavior and flame resistance compared to other polymer systems (they do not burn or release noxious chemicals). Incorporating aerogel material into the polyimide foam as described by this technology creates a composite that has been demonstrated to provide additional performance gains, including 25% lower thermal conductivity with no compromise of the structural integrity and high-temperature behavior of the base polyimide foam. The structural properties of Aerofoam are variable based on its
formulation, and it can be used in numerous rigid and flexible foams of varying densities.
Aerofoam has a number of potential commercial applications, including construction, consumer appliances, transportation, electronics, healthcare, and industrial equipment. In addition, these high-performance materials may prove useful in applications that require insulation that can withstand harsh environments, including process piping, tanks for transporting and storing hot or cold fluids, ship and boat building, and aerospace applications.
power generation and storage

High-Performance, Lightweight, Easy-to-Fabricate Heat Exchanger
Researchers at JPL have developed, built, and tested an innovative heat exchanger that offers reduced thermal expansion, increased structural strength, low pressure drop, and improved thermal performance while lowering the weight associated with typical heat exchangers. This innovation would benefit the commercial thermoelectric generator, aircraft, and industrial processing (i.e., glass, steel, petrochemical, cement, aluminum) industries by improving energy management/efficiency, reducing carbon dioxide emissions, and increasing system durability due to the reduced stress from thermal expansion.
<b>The Problem</b>
Thermoelectric generator systems require high-performance hot-side and cold-side heat exchangers to provide the temperature differential needed to transfer thermal energy while withstanding temperatures up to 650 °C. Because the hot-side heat exchangers must have a high heat flux, they are often made of metals such as stainless steel or Inconel<sup>®</sup> alloys. Although these materials can operate at high temperatures, resist corrosion, and are chemically stable, they also have several drawbacks: (1) Their lower thermal conductivity negatively affects their thermal performance. (2) Their higher thermal expansion leads to stresses that compromise system structural integrity. (3) Their high mass/volume reduces the power density of generator systems into which they are integrated. As a result, they are difficult to integrate into viable energy recovery systems. They also make the systems unreliable, non-durable, and susceptible to failures caused by thermal-structural expansion.
<b>The Solution</b>
JPL researchers chose to replace the metal in traditional heat exchangers with graphite, which offers an improved conductivity-to-density ratio in thermal applications as well as a low coefficient of thermal expansion. In addition, they used a mini-channel design to further increase thermal performance. Combining more advanced materials with the innovative thermal design has yielded significant improvements in performance. For example, a 200-cm<sup>3</sup>, 128-g version of JPL's exchanger successfully transported 1,100 W from exhaust at nearly 550 °C with approximately 20 W/cm<sup>2</sup> thermal flux and a pressure drop of only 0.066 psi.
JPL's technology combines lightweight, high-strength graphite material with a mini-channel design that offers high thermal performance. Further development and testing are underway.
<em>Inconel is a registered trademark of Special Materials Corporation.</em>
manufacturing
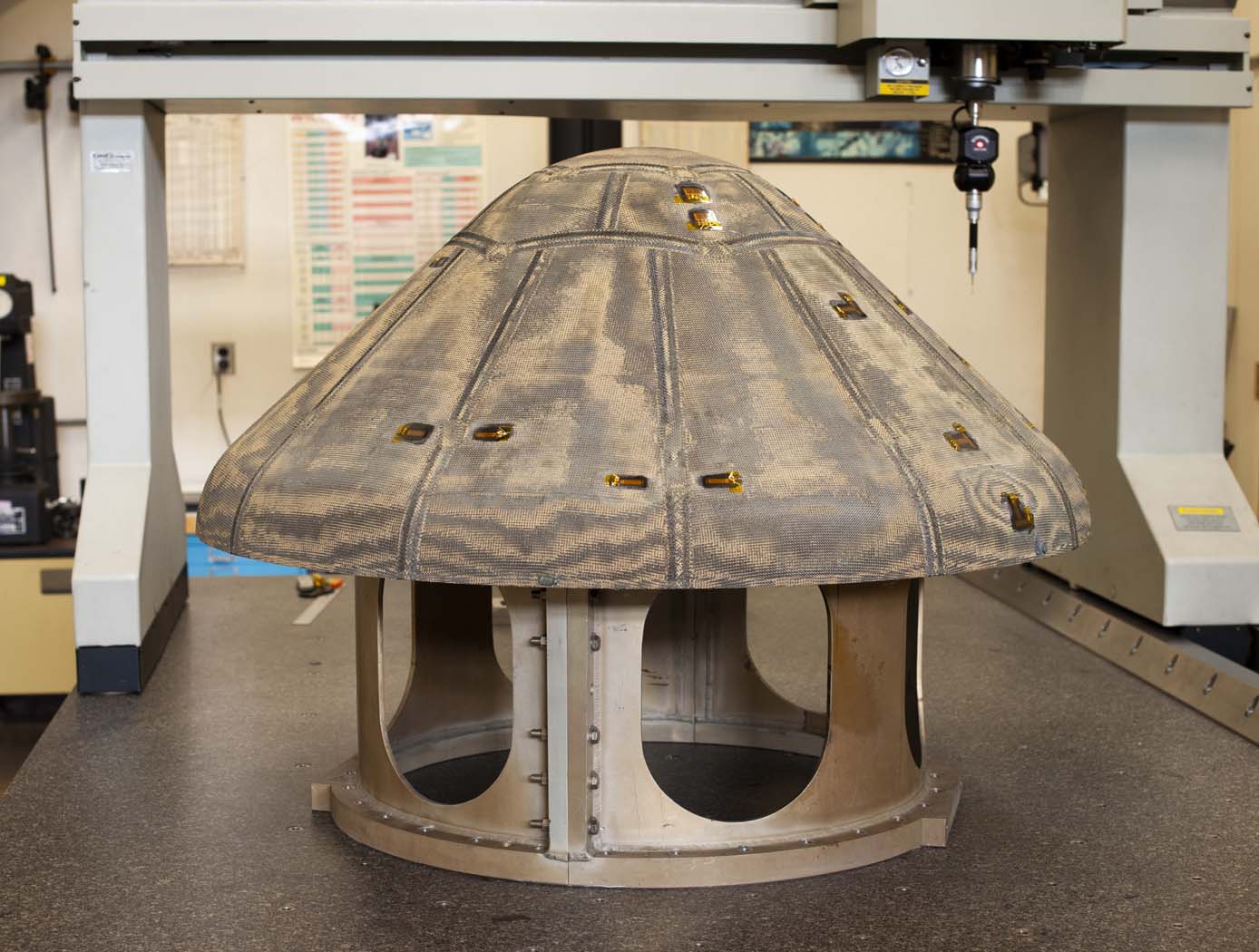
Woven Thermal Protection System
Going farther, faster and hotter in space means innovating how NASA constructs the materials used for heat shields. For HEEET, this results in the use of dual-layer, three-dimensional, woven materials capable of reducing entry loads and lowering the mass of heat shields by up to 40%. The outer layer, exposed to a harsh environment during atmospheric entry, consists of a fine, dense weave using carbon yarns. The inner layer is a low-density, thermally insulating weave consisting of a special yarn that blends together carbon and flame-resistant phenolic materials. Heat shield designers can adjust the thickness of the inner layer to keep temperatures low enough to protect against the extreme heat of entering an atmosphere, allowing the heat shield to be bonded onto the structure of the spacecraft itself. The outer and inner layers are woven together in three dimensions, mechanically interlocking them so they cannot come apart. To create this material, manufacturers employ a 3-D weaving process that is similar to that used to weave a 2-D cloth or a rug. For HEEET, computer-controlled looms precisely place the yarns to make this kind of complex three-dimensional weave possible. The materials are woven into flat panels that are formed to fit the shape of the capsule forebody. Then the panels are infused with a low-density version of phenolic material that holds the yarns together and fills the space between them in the weave, resulting in a sturdy final structure. As the size of each finished piece of HEEET material is limited by the size of the loom used to weave the material, the HEEET heat shield is made out of a series of tiles. At the points where each tile connects, the gaps are filled through inventive designs to bond the tiles together.
materials and coatings

Conductive Carbon Fiber Polymer Composite
The new composite developed by NASA incorporates PGS and CNTs to enhance its thermal conductivity while preserving the mechanical properties of the underlying carbon fiber polymer composite. NASA has also improved the composite manufacturing process to ensure better thermal conductivity not only on the surface, but also through the thickness of the material. This was achieved by adding perforations that enable the additives to spread through the composite.
The process for developing this innovative, highly thermally conductive hybrid carbon fiber polymer composite involves several steps. Firstly, a CNT-doped polymer resin is prepared to improve the matrix's thermal conductivity, which is then infused into a carbon fiber fabric. Secondly, PGS is treated to enhance its mechanical interface with the composite. Thirdly, perforation is done on the pyrolytic graphite sheet to improve the thermal conductivity through the thickness of the material by allowing CNT-doped resin to flow and better interlaminar mechanical strength. Finally, the layup of PGS and CNT-CF polymer is optimized.
Initial testing of the composite has shown significant increases in thermal conductivity compared to typical carbon fiber composites, with a more than tenfold increase. The composite also has higher thermal conductivity than aluminum alloys, with more than twice the thermal conductivity of the Aluminum 6061 typically used in the aerospace industry. For this new material, NASA has completed a proof-of-concept demonstration and work continues to use the material in a heat exchanger system and further characterize the properties including longevity and radiation impact analysis.
Materials and Coatings

Advanced Materials for Electronics Insulation
Many researchers have attempted to use polymer-ceramic composites to improve the thermal and dielectric performance of polymer insulation for high voltage, high temperature electronics. However, using composite materials has been challenging due to manufacturing issues like incomplete mixing, inhomogeneous properties, and void formation. Here, NASA has developed a method of preparing and extruding polymer-ceramic composites that results in high-quality, flexible composite ribbons.
To achieve this, pellets of a thermoplastic (e.g., polyphenylsulfone or PPSU) are coated with an additive then mixed with particles of a ceramic (e.g., boron nitride or BN) as shown in the image below. After mixing the coated polymer with the ceramic particles, the blended material was processed into ribbons or films by twin-screw extrusion. The resulting ribbons are highly flexible, well-mixed, and void free, enabled by the coated additive and by using a particle mixture of micronized BN and nanoparticles of hexagonal BN (hBN).
The polymer-ceramic composite showed tunable dielectric and thermal properties depending on the exact processing method and composite makeup. Compared to the base polymer material, the composite ribbons showed comparable or improved dielectric properties and enhanced thermal conductivity, allowing the composite to be used as electrical insulation in high-power, high-temperature conditions.
The related patent is now available to license. Please note that NASA does not manufacturer products itself for commercial sale.
Optics
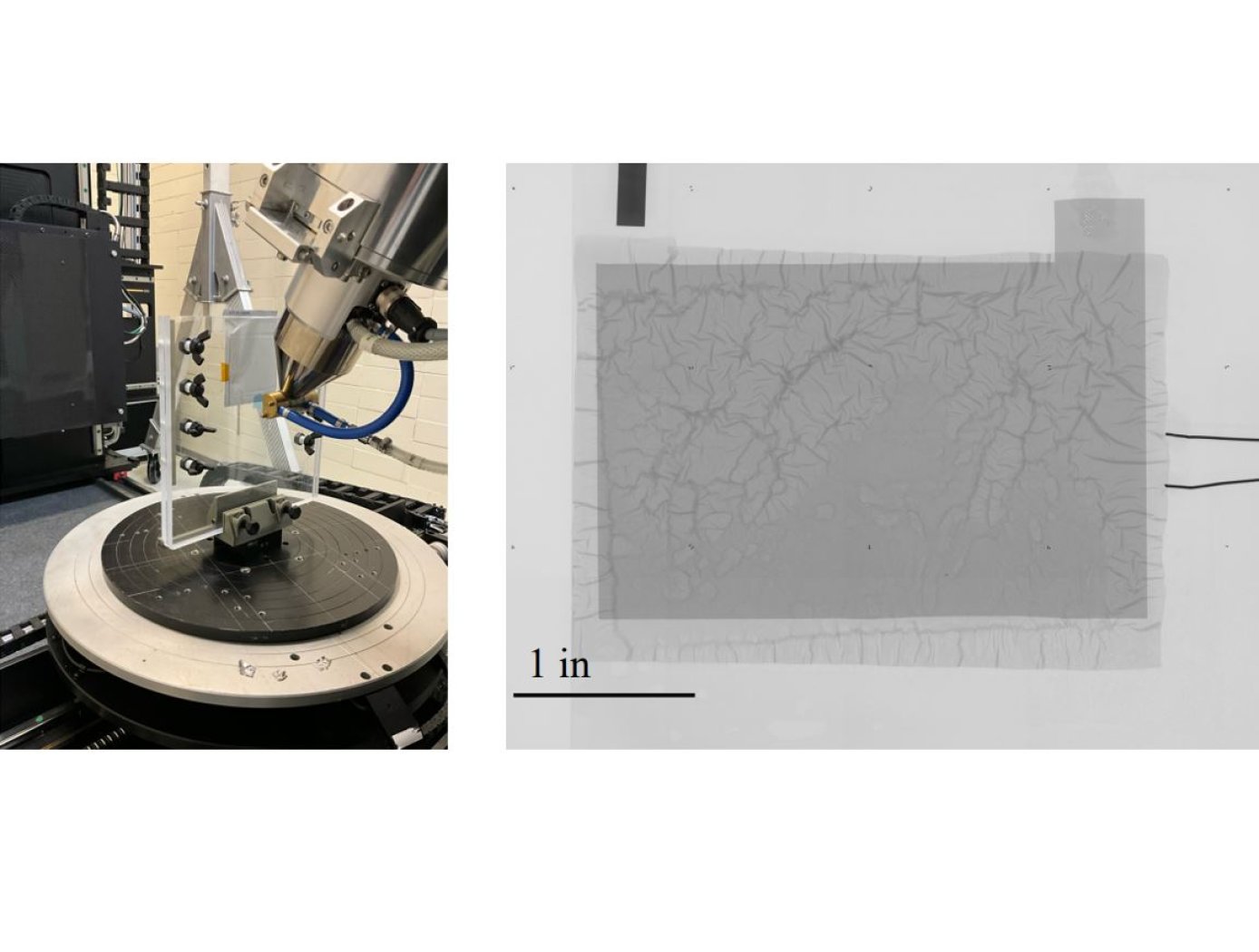
Fingerprinting for Rapid Battery Inspection
The technology utilizes photopolymer droplets (invisible to the digital radiograph) with embedded radiopaque fragments to create randomized fingerprints on battery samples. The droplets are deposited using a jig (see figure on right) that precisely positions samples. Then, at different points during battery R&D testing or use, digital radiography imaging with micron-level resolution can be performed.
The high-resolution imaging required to detect dendrite formation requires images to be collected in multiple “tiles” as shown below. The randomized fingerprints uniquely identify relative positioning of these tiles, allowing rapid assembly of composite high-resolution images from multiple tiles.
This same composite creation process can be used for images taken at a series of points in time during testing, and background subtraction can be applied to efficiently compare how the battery is changing over successive charge/discharge cycles to identify dendrite formation. This inspection technique is proven effective for thin-film pouch cell prototypes at NASA, and it works well at the lowest available x-ray energy level (limiting impact on the samples). The Fingerprinting for Rapid Battery Inspection technology is available for patent licensing.
Materials and Coatings
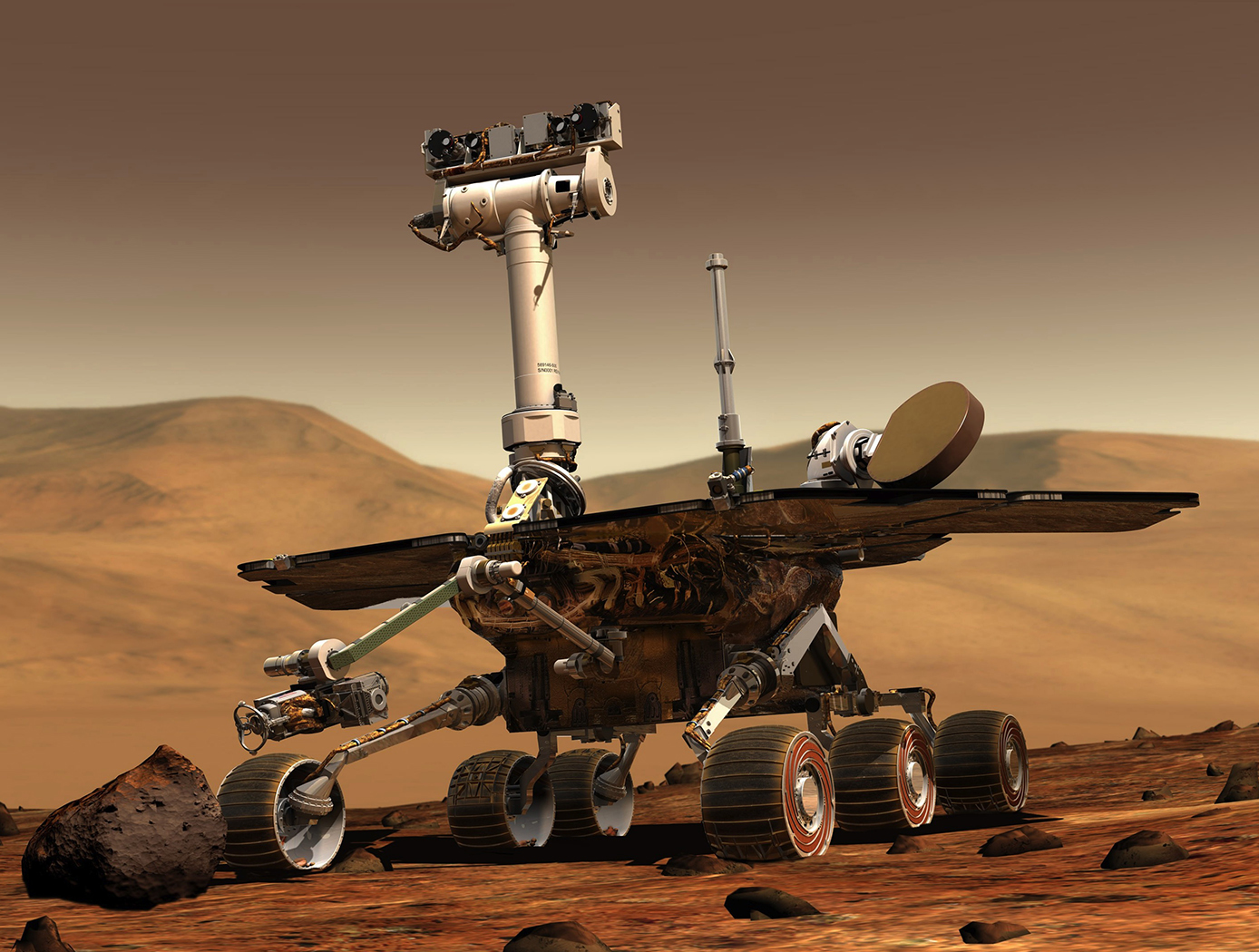
New Methods in Preparing and Purifying Nanomaterials
Sometimes called white graphite, affordable and plentiful hBN possesses the same kind of layered molecular structure as graphite. In graphite, this structure has allowed next-generation nanomaterials like carbon nanotubes and graphene to be produced. With hBN, however, the process of converting the substance into boron nitride nanotubes (BNNT) has been too difficult to yield commercial quantities. Glenn innovators have created several new methods that could enable greater adoption of this unique nanomaterial. In the initial stage, the starter reactant is mixed with a selected set of chemicals (a metal chloride, for example) and an activation agent (such as sodium fluoride). This mixture causes hBN to become less resistant to intercalation. The intercalated product can then be exfoliated by heating the material in air, and giving the material a final rinse with a liquid-phase ferric chloride salt to dissolve any embedded impurities without damaging its internal structure. These efficiently exfoliated nanomaterials can be used to form advanced composite materials (e.g., layered with aluminum oxide to form hBN/alumina ceramic composites). Nanomaterials fabricated from hBN can also take advantage of the material's unique combination of being an electrical insulator with high thermal conductivity for applications ranging from microelectronics to energy harvesting. Glenn's innovations have enabled a significantly improved matrix composite material with the potential to make a significant impact on the commercial materials market.
Power Generation and Storage
Nail Penetration Adapter for
Li-Ion Battery Testing
NASA’s pneumatic nail penetration trigger system embodies a fast and consistent nail penetration and retraction tool that tests battery cells within various steel and mylar enclosures. It is operated remotely with electric power and shop air pressure inputs. The trigger system is controlled by a solenoid valve and drives a nail to a set distance at 100 m/s for a precise and repeatable penetration injury to a battery cell.
Contributing to the trigger system’s precision and repeatability is the nail penetration adapter that aligns the battery cell test enclosure with the trigger system and guides the nail by its internal barrel. The tip of the adapter threads into a variety of NASA battery cell test enclosures and provides an internal seating stop to ensure a proper targeting depth. On the actuator side of the adapter, two quick-release pins connect the adapter to the actuator mount which is bolted to the actuator subassembly. Removal of these pins readily allow for separation of the nail penetration adapter from the actuator mount and subassembly. During a TR event, the adapter is also designed to prevent flames, sparks, and ejecta from traveling into the actuator subassembly.
While the versatile nail penetration adapter was originally configured to interface with NASA’s Fractional Thermal Runaway Calorimeter (FTRC), a blast plate test platform (BPTP), or cell enclosure for passive propagation resistant (PPR) methods, the adapter could be modified for commercial use with other battery testing systems. Companies interested in this technology may include those seeking to improve the safety of Li-ion battery cells and packs along with vertically integrated companies performing in-house TR tests during development of electrified systems like electric vehicles (EVs), electric vertical takeoff and landing (eVTOLs) vehicles, and electronic spacecraft components.
Optics
Ruggedized Infrared Camera
This new technology applies NASA engineering to a FLIR Systems Boson® Model No. 640 to enable a robust IR camera for use in space and other extreme applications. Enhancements to the standard Boson® platform include a ruggedized housing, connector, and interface. The Boson® is a COTS small, uncooled, IR camera based on microbolometer technology and operates in the long-wave infrared (LWIR) portion of the IR spectrum. It is available with several lens configurations. NASA's modifications allow the IR camera to survive launch conditions and improve heat removal for space-based (vacuum) operation. The design includes a custom housing to secure the camera core along with a lens clamp to maintain a tight lens-core connection during high vibration launch conditions. The housing also provides additional conductive cooling for the camera components allowing operation in a vacuum environment. A custom printed circuit board (PCB) in the housing allows for a USB connection using a military standard (MIL-STD) miniaturized locking connector instead of the standard USB type C connector. The system maintains the USB standard protocol for easy compatibility and "plug-and-play" operation.
Sensors
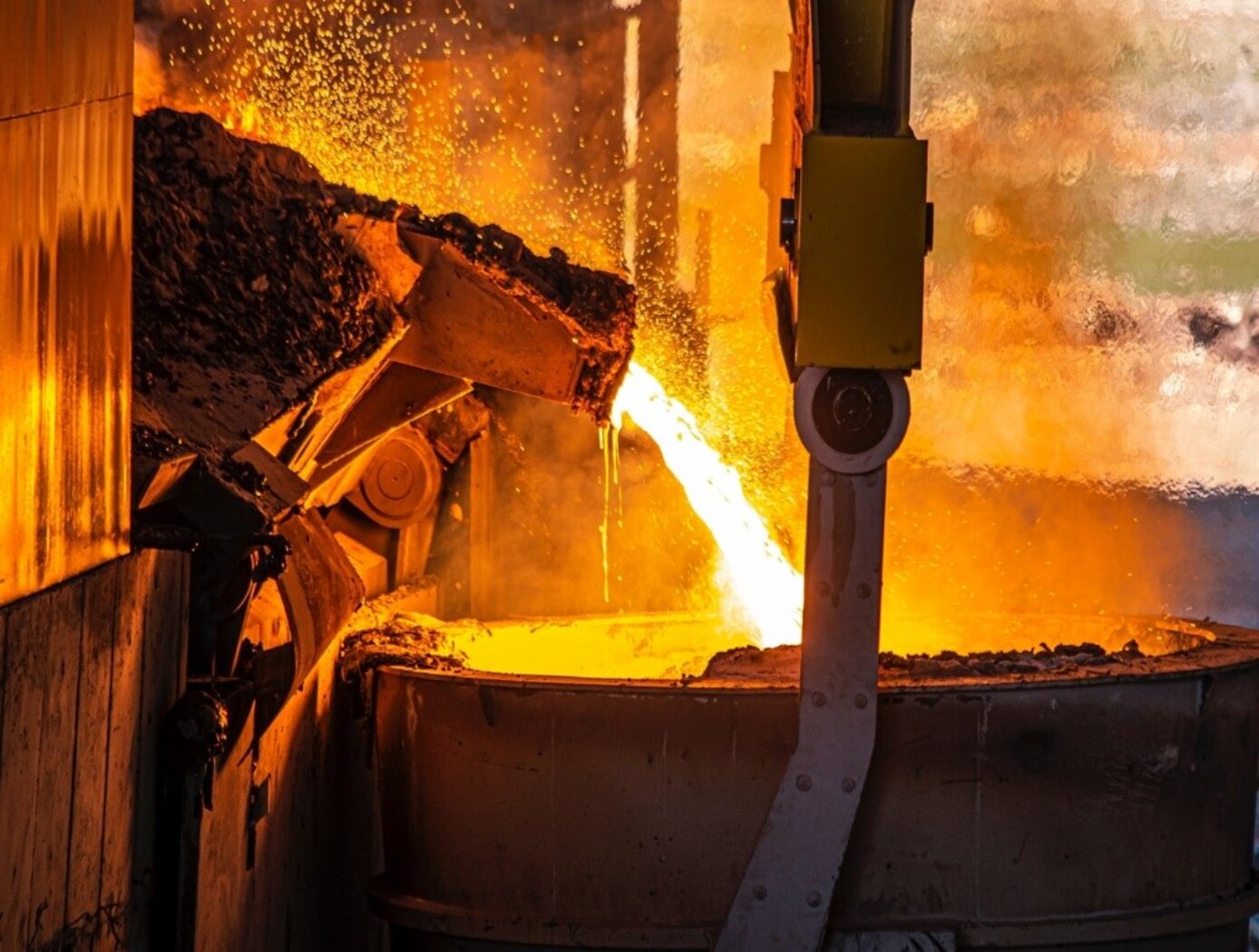
Multi-Spectral Imaging Pyrometer
This NASA technology transforms a conventional infrared (IR) imaging system into a multi-wavelength imaging pyrometer using a tunable optical filter. The actively tunable optical filter is based on an exotic phase-change material (PCM) which exhibits a large reversible refractive index shift through an applied energetic stimulus. This change is non-volatile, and no additional energy is required to maintain its state once set. The filter is placed between the scene and the imaging sensor and switched between user selected center-wavelengths to create a series of single-wavelength, monochromatic, two-dimensional images. At the pixel level, the intensity values of these monochromatic images represent the wavelength-dependent, blackbody energy emitted by the object due to its temperature. Ratioing the measured spectral irradiance for each wavelength yields emissivity-independent temperature data at each pixel. The filter’s Center Wavelength (CWL) and Full Width Half Maximum (FWHM), which are related to the quality factor (Q) of the filter, are actively tunable on the order of nanoseconds-microseconds (GHz-MHz). This behavior is electronically controlled and can be operated time-sequentially (on a nanosecond time scale) in the control electronics, a capability not possible with conventional optical filtering technologies.