Search
mechanical and fluid systems
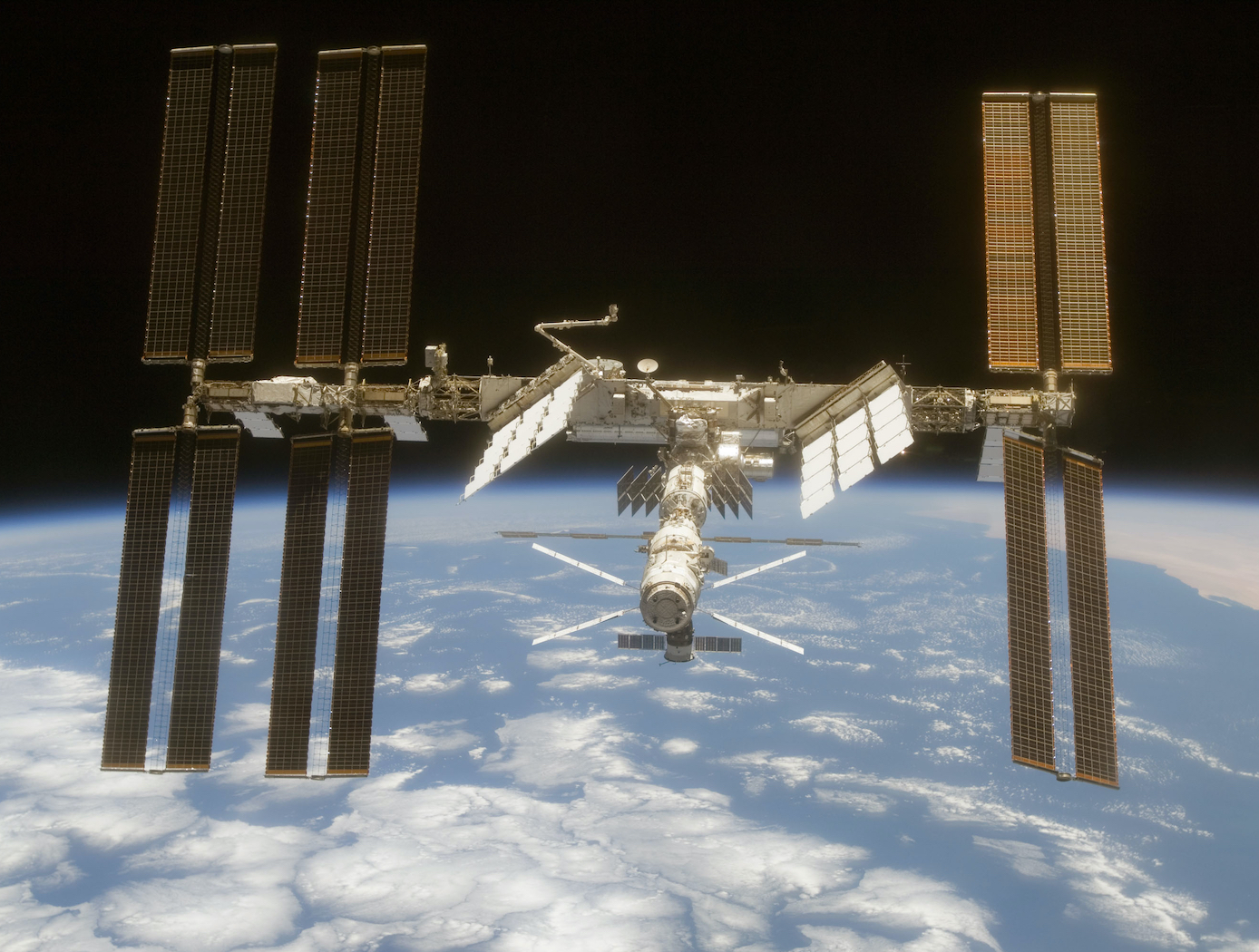
Liquid Sorbent Carbon Dioxide Removal System
NASA's Liquid Sorbent Carbon Dioxide Removal System was designed as an alternative to the current CO2 removal technology used on the International Space Station (ISS), which uses solid zeolite media that is prone to dusting, has a low absorption capacity, and requires high regeneration temperatures and frequent maintenance. Motivated by CO2 removal systems on submarines, NASA innovators began investigating the use of liquid sorbents. Liquid sorbents have a capacity four times greater than solid zeolites, require low regeneration temperature, and need fewer unreliable moving mechanical parts than solid based systems. While submarine CO2 scrubbers spray an adsorbing chemical directly into the air stream and allow the liquid to settle, NASA's new system uses a capillary driven 3D printed microchannel direct air/liquid contactor in a closed loop system. The Liquid Sorbent Carbon Dioxide Removal System is robust and reliable, while being low in weight, volume, and power requirements. The system is capable of reaching equilibrium when the liquid sorbent surface is being regenerated at a rate equal to the rate of absorption into the liquid.
instrumentation
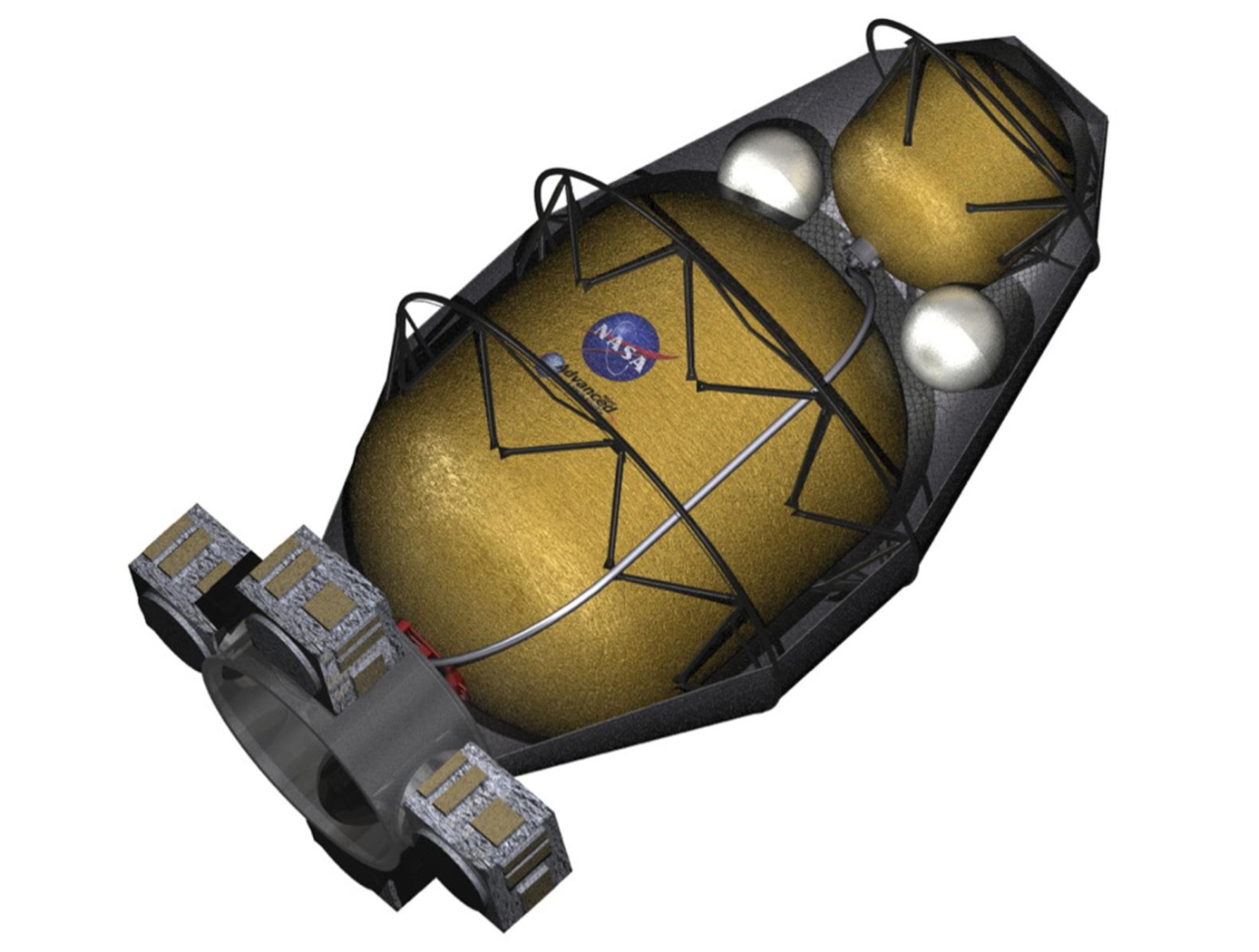
Capacitive Micro-Gravity Fluid Mass Gauge
The capacitive micro-gravity fluid mass gauge with spatial regularization is a sensor that can be outfitted to propellant vessels and can provide a determination of the mass of liquid and gas inside the vessel volume with a determinable level of accuracy. The sensor consists of 1) a number of discrete electrodes that are installed to the inner surface of the vessel wall, 2) signal generating, digitizing, signal conditioning, and general support (e.g., power supply) electronics, 3) electrical connections between the electrodes and the electronics, and 4) the algorithm used to turn the set of capacitance measurements (i.e., the capacitance matrix) into a volume fraction. The electronics generate and apply a sinusoid to a single electrode, and then the electronics measure the charge on all other electrodes. Capacitance is simply the charge divided by the voltage. This is repeated for all electrodes, without repeating duplicates. For a vessel with a fixed volume, the volume fraction can be converted to the mass fraction using the Ideal Gas Law so long as the fluid constituents, temperature, and pressure are known.
Power Generation and Storage
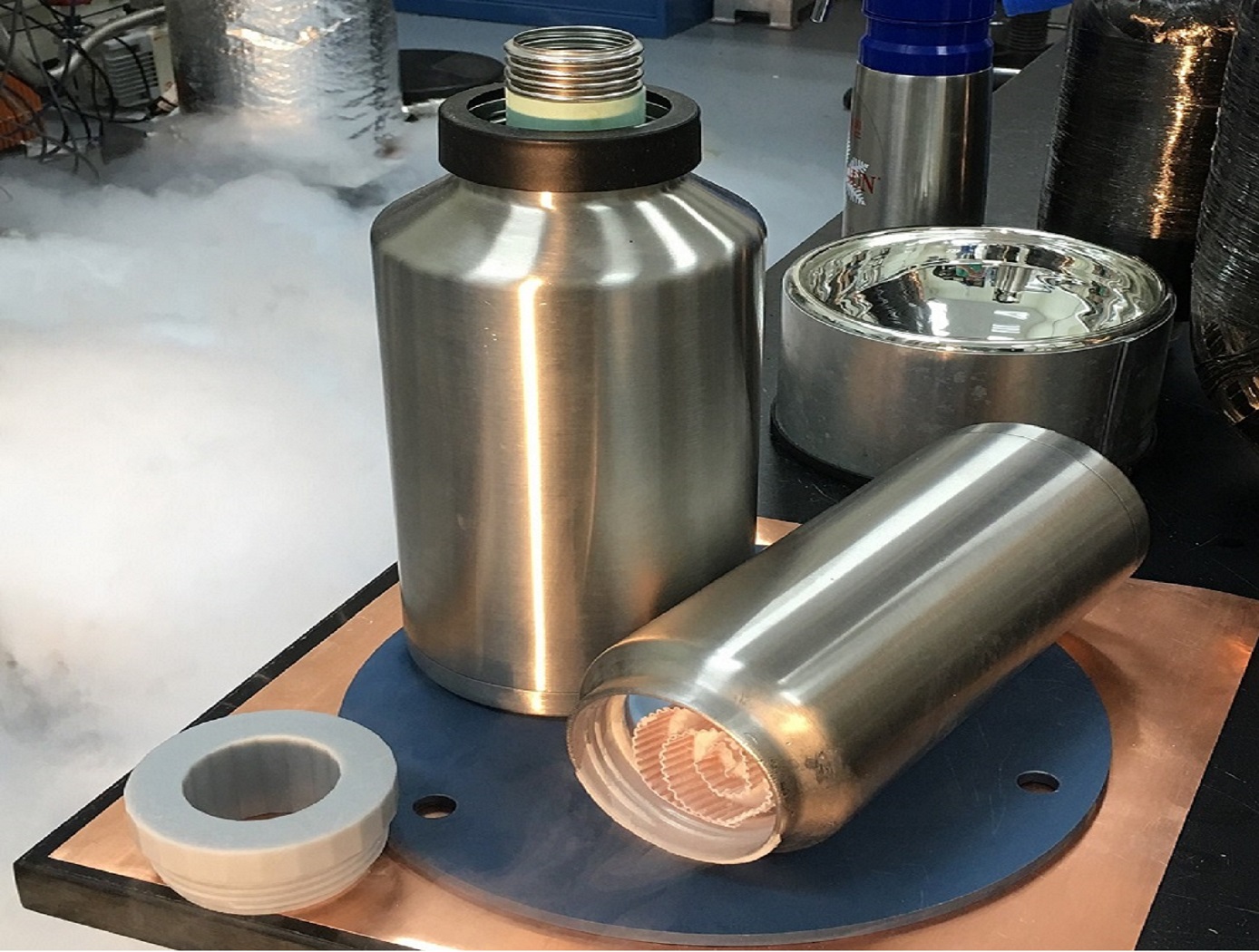
Cryogenic Flux Capacitor
Storage and transfer of fluid commodities such as oxygen, hydrogen, natural gas, nitrogen, argon, etc. is an absolute necessity in virtually every industry on Earth. These fluids are typically contained in one of two ways; as low pressure, cryogenic liquids, or as a high pressure gases. Energy storage is not useful unless the energy can be practically obtained ("un-stored") as needed. Here the goal is to store as many fluid molecules as possible in the smallest, lightest weight volume possible; and to supply ("un-store") those molecules on demand as needed in the end-use application. The CFC concept addresses this dual storage/usage problem with an elegant charging/discharging design approach.
The CFC's packaging is ingeniously designed, tightly packing aerogel composite materials within a container allows for a greater amount of storage media to be packed densely and strategically. An integrated conductive membrane also acts as a highly effective heat exchanger that easily distributes heat through the entire container to discharge the CFC quickly, it can also be interfaced to a cooling source for convenient system charging; this feature also allows the fluid to easily saturate the container for fast charging. Additionally, the unit can be charged either with cryogenic liquid or from an ambient temperature gas supply, depending on the desired manner of refrigeration. Finally, the heater integration system offers two promising methods, both of which have been fabricated and tested, to evenly distribute heat throughout the entire core, both axially and radially.
NASA engineers also applied the CFC to a Cryogenic Oxygen Storage Module to store oxygen in solid-state form and deliver it as a gas to an end-use environmental control and/or life support system. The Module can scrub out nuisance or containment gases such as carbon dioxide and/or water vapor in conjunction with supplying oxygen, forming a synergistic system when used in a closed-loop application. The combination of these capabilities to work simultaneously may allow for reduced system volume, mass, complexity, and cost of a breathing device.
mechanical and fluid systems
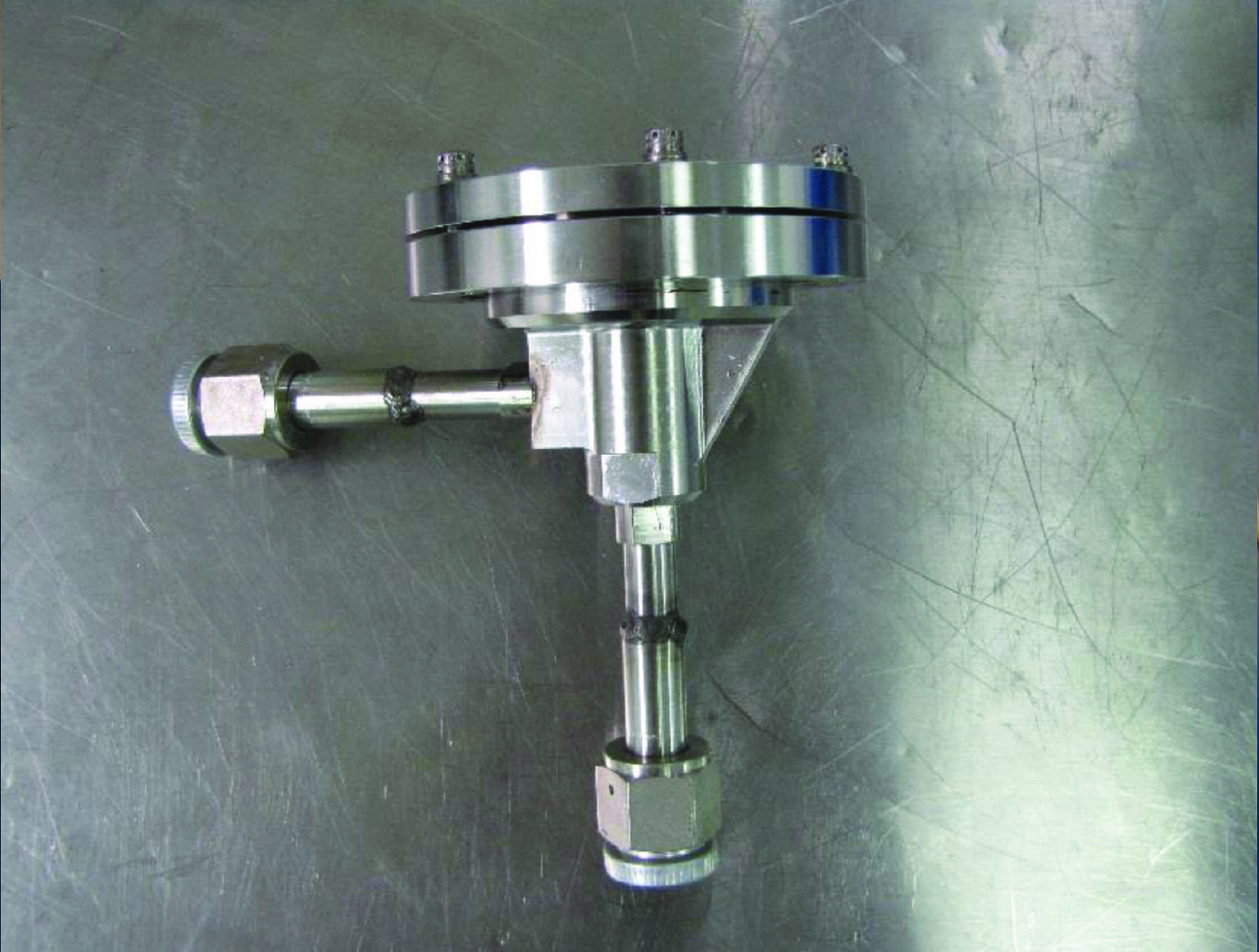
Pilot Assisted Check Valve for Low Pressure Applications
Check valves are traditionally designed as a simple poppet/spring system where the spring is designed to equal the force created from the sealing area of the valve seat multiplied by the cracking pressure. Since the valve seat diameter in these types of valves are relatively small, less than 0.5 inches diameter, a low cracking pressure required for back pressure relief devices results in a low spring preload. When sealing in the reverse direction, the typical 20 psid storage pressure of the cryogenic fluid is not enough pressure force to provide adequate sealing stress. To better control the cracking pressure and sealing force, a bellows mechanism was added to a poppet check valve (see Figure 2). The bellows serves as a reference pressure gauge; once the targeted pressure differential is reached, the bellows compresses and snaps the valve open. Prior to reaching the desired crack pressure differential, the bellows diaphragm is fully expanded, providing sufficient seal forces to prevent valve flow (including reverse flow) and undesired internal leakage. Room temperature testing of cracking pressure, full flow pressure, and flow capacity all showed improvements. The overall results of the test proved to be 10-20 times greater than conventional check valves with no internal leakage at three different pressure differentials.
mechanical and fluid systems
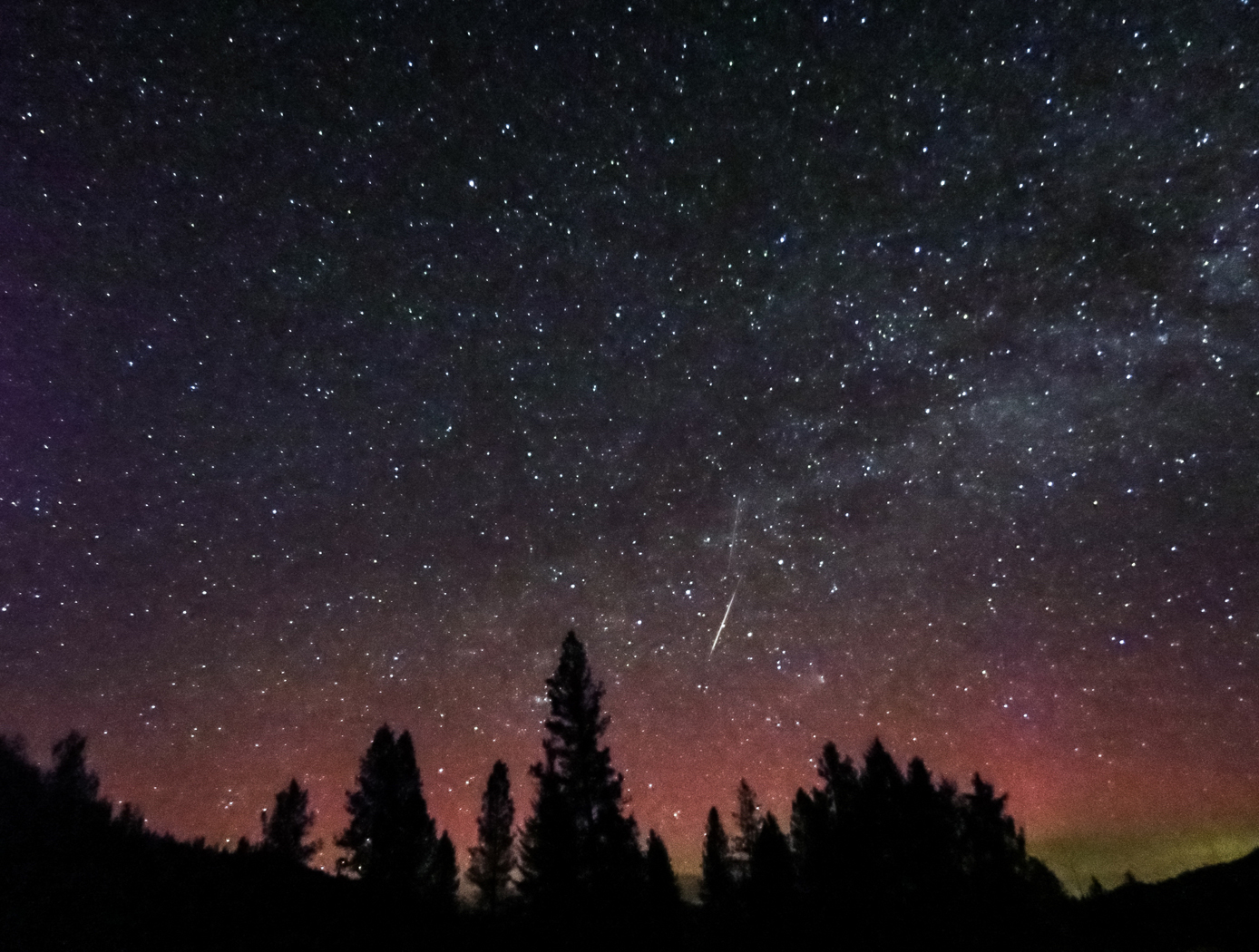
Normally-closed (NC) Zero Leak Valve
The valve consists of two major sub-assemblies: the
actuator and the flow cavity. The actuator is preloaded to 1,250 N by adjusting the preload bolt, pressing the Terfenol-D against the now-deflected belleville springs. When actuation is needed, either solenoid coil is charged in a pulsed mode, causing magnetostriction or elongation in the Terfenol-D which deflects the belleville spring stack, supplying an increasing load to the stem until the parent metal seal is fractured. Once fractured, the spring inside the bellows drives the bellows base downward, onto a raised boss at the top of the fracture plate. When fracture has occurred, the stem and its spring stack is left, separated from the actuator column. The Terfenol-D is unloaded and returns to its original length. The valve remains open due to the spring inside the bellows.
materials and coatings
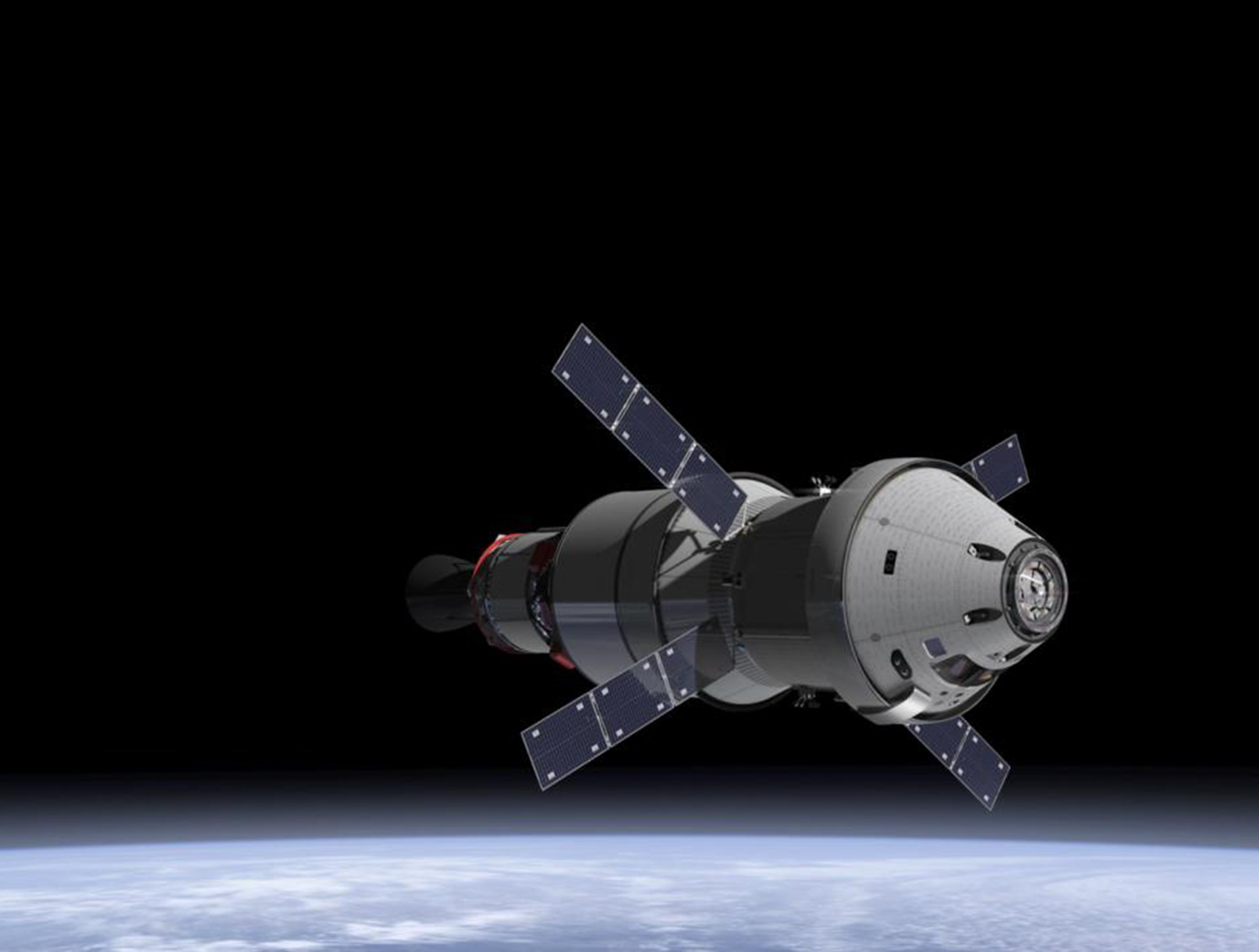
Advanced Protective Coatings for Graphite-Based Nuclear Propulsion Fuel Elements
To protect the graphite (Gr)-based substrates in a nuclear systems fuel elements from hot hydrogen attacks, earlier researchers developed a method to deposit (via chemical vapor deposition) a protective niobium carbide (NbC) or zirconium carbide (ZrC) coating in the inner cooling channels of the fuel elements through which the hydrogen propellant flows. Unfortunately, the significant difference in the coefficients of thermal expansion (CTE) between the Gr-based substrate and the ZrC coating leads to debonding at intermediate temperatures, thereby exposing the substrate to hot hydrogen attack despite the NbC or ZrC coating. Innovators at Glenn have proposed a solution to this problem by introducing additional layers of compliant metallic coatings to accommodate the differences in CTE between the ZrC and the Gr-substrate,thereby potentially increasing life and durability. In this configuration,the innermost layer is composed of molybdenum carbide (Mo<sub>2</sub>C), and additional outer layers are made of molybdenum (Mo) and niobium (Nb) layers. The MoC acts as a diffusion barrier to minimize the diffusion of carbon into the refractory metal layers and the diffusion of Mo or Nb into the Gr-based substrate. The Mo layer is deposited on top of the Mo<sub>2</sub>C layer. A Nb layer is deposited on the Mo layer with the ZrC forming the outside layer of the coating. A thin Mo layer on the ZrC helps to seal the cracks on the ZrC and acts a diffusion barrier to hydrogen diffusion into the coating.The Mo and Nb layers are compliant so that differences in the thermal expansion of ZrC and other layers can be accommodated without significant debonding or cracking. They also act as additional diffusion barriers to hydrogen diffusion towards the Gr-substrate. Overall, Glenn's pioneering use of layered coatings for these components will potentially increase the durability and performance of nuclear propulsion rockets.
mechanical and fluid systems
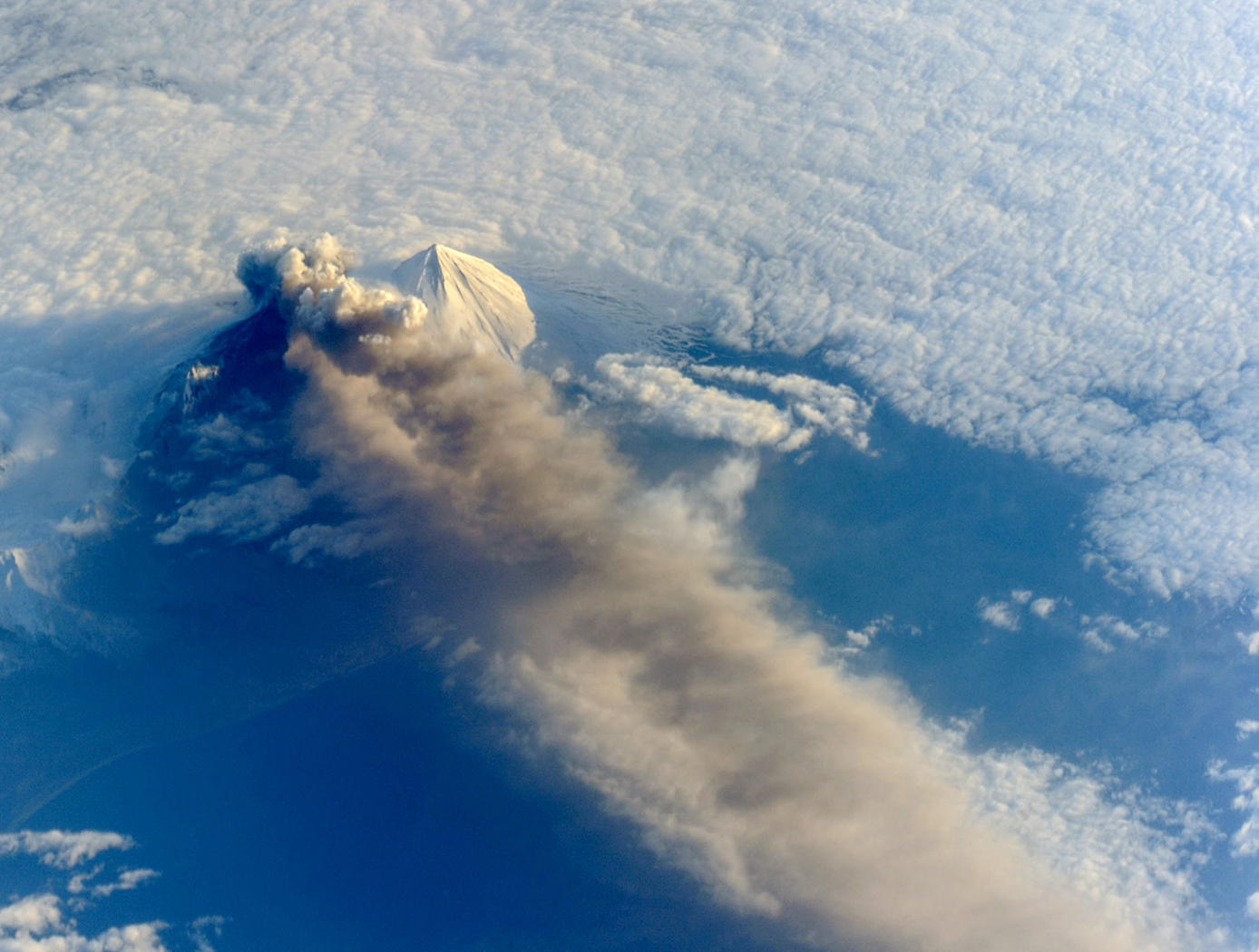
Nitinol-Actuated Normally Open Valve Assembly (NOVA)
The nitinol-actuated Normally Open Valve Assembly (NOVA) is a type of zero-leak permanent isolation valve designed for liquid propellant service on in-space propulsion systems with operating pressures less than or equal to 500 psia. The NOVA is a drop-in replacement for the currently used pyrovalve. Prior to actuation, the valve allows propellant flow with a pressure drop of < 3 psi at a flow rate of 0.15 kg/s. A compressed piece of nitinol inside the valve is heated once actuation is desired, causing the nitinol to recover to its origin shape. This recovery closes the valve, creating a leak-tight seal. The valve is compatible with all storable propellants.
mechanical and fluid systems
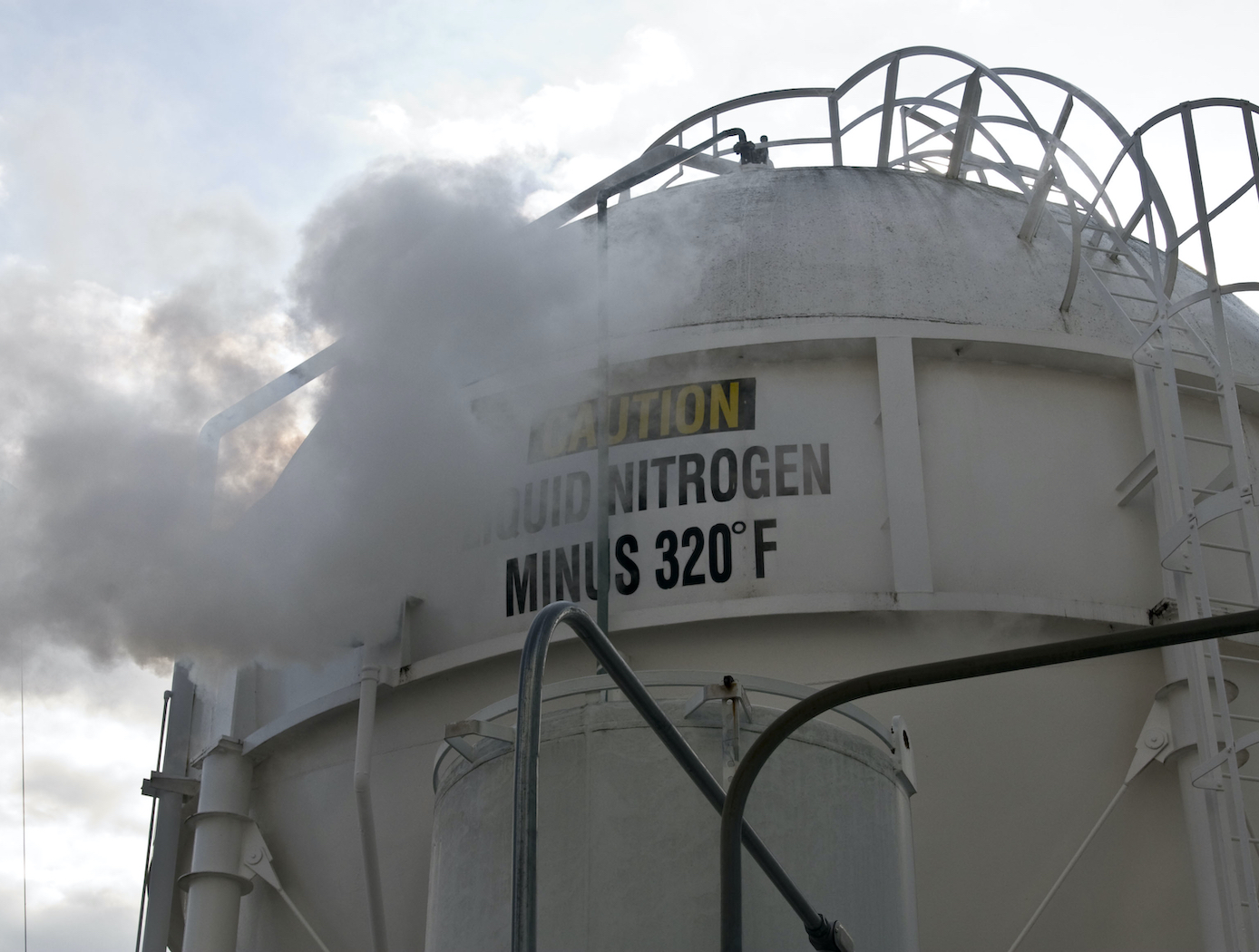
3D-Printed Injector for Cryogenic Fluid Management
NASA's TVS Augmented Injector includes an internal heat exchanger, a fluid injector spray head, and an external surface condensation heat exchanger - all combined with multiple intertwined flow paths containing liquid, two-phase, and gaseous working fluid. The TVS provides a source of coolant to the injector, which chills the incoming fluid flow. This cooled flow promotes condensation of the tank ullage dropping pressure and maintains incoming fluid flow. The system eliminates the potential for a stalled fill condition and reduces tank pressure during cryogenic fluid transfer. During fill operations, the tank vent can be closed early in the process before fluid is introduced, and, in some cases, the tank vent may not even need to be opened. Furthermore, the TVS Augmented Injector can remove sufficient thermal energy to reach a 100% liquid level in the receiver tank. A cryo-cooler can be used in place the TVS flow circuit for a zero-loss system. The TVS Augmented Injector couples internal fluid flow cooling and external surface ullage gas condensation into a single, compact package that can be mounted to small tank flanges for minimal impact insertion into any vessel. The injector is printed as one part using additive manufacturing, resulting in part count reduction, improved reproducibility, shorter lead times, and reduced cost compared to conventional approaches.
The injector may be of particular interest in applications where cryogenic fluid is expensive, fluid loss through vents is problematic, and/or achieving high filling levels would be helpful. The injector can benefit typical cryogenic fluid transfer between containers or, alternatively, can serve as a tank pressure control device for long-term storage using a fluid recirculation system that pumps fluid through the injector and sprays cooled liquid back into the tank. Additionally, where ISRU processes are employed, the injector can be used to liquefy incoming propellant streams.
manufacturing
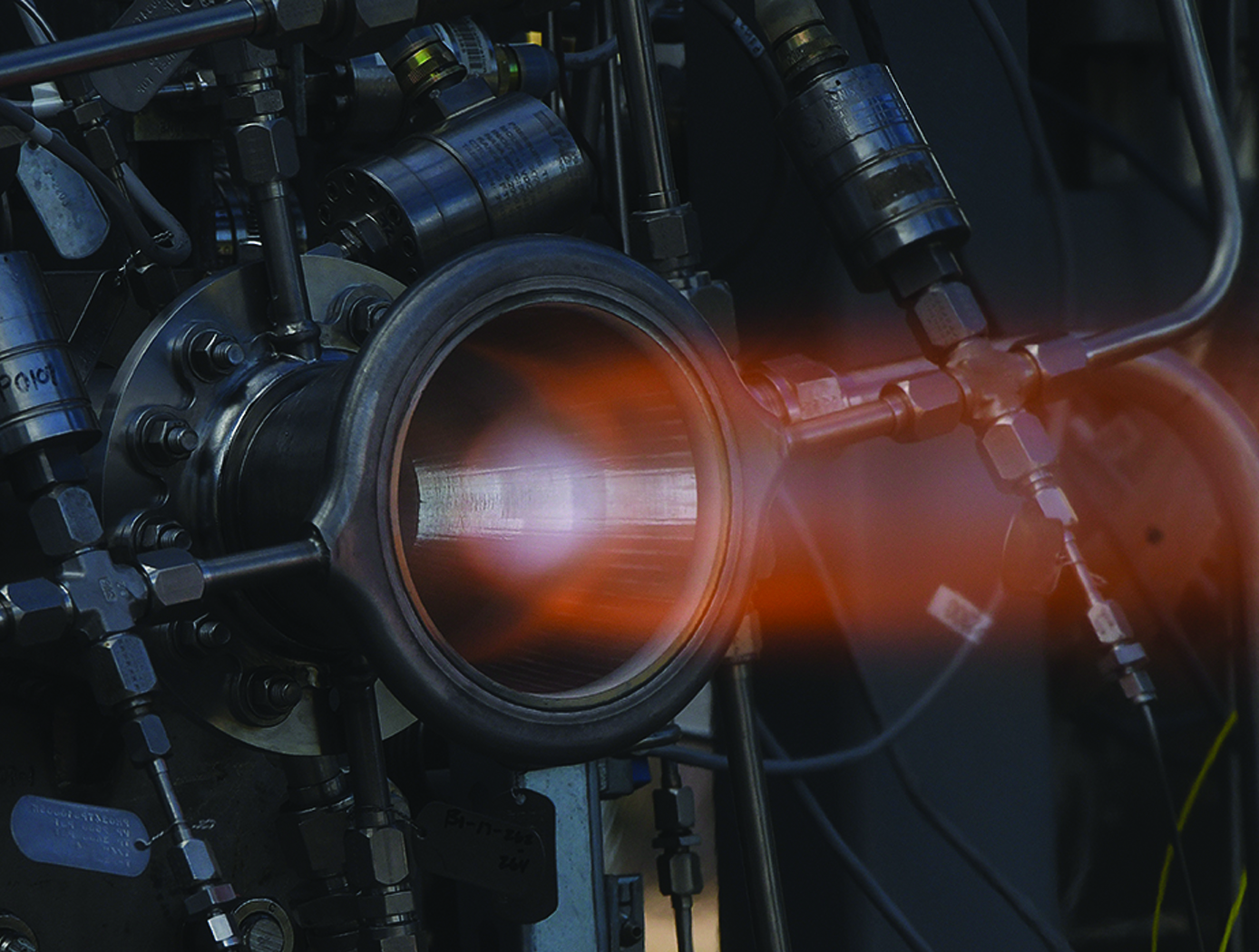
Cladding and Freeform Deposition for Coolant Channel Closeout
LWDC technology enables an improved channel wall nozzle with an outer liner that is fused to the inner liner to contain the coolant. It is an additive manufacturing technology that builds upon large-scale cladding techniques that have been used for many years in the oil and gas industry and in the repair industry for aerospace components. LWDC leverages wire freeform laser deposition to create features in place and to seal the coolant channels. It enables bimetallic components such as an internal copper liner with a superalloy jacket. LWDC begins when a fabricated liner made from one material, Material #1, is cladded with an interim Material #2 that sets up the base structure for channel slotting. A robotic and wire-based fused additive welding system creates a freeform shell on the outside of the liner. Building up from the base, the rotating weld head spools a bead of wire, closing out the coolant channels as the laser traverses circumferentially around the slotted liner. This creates a joint at the interface of the two materials that is reliable and repeatable. The LWDC wire and laser process is continued for each layer until the slotted liner is fully closed out without the need for any filler internal to the coolant channels. The micrograph on the left shows the quality of the bond at the interface of the channel edge and the closeout layer; on the right is a copper channel closed out with stainless.
sensors
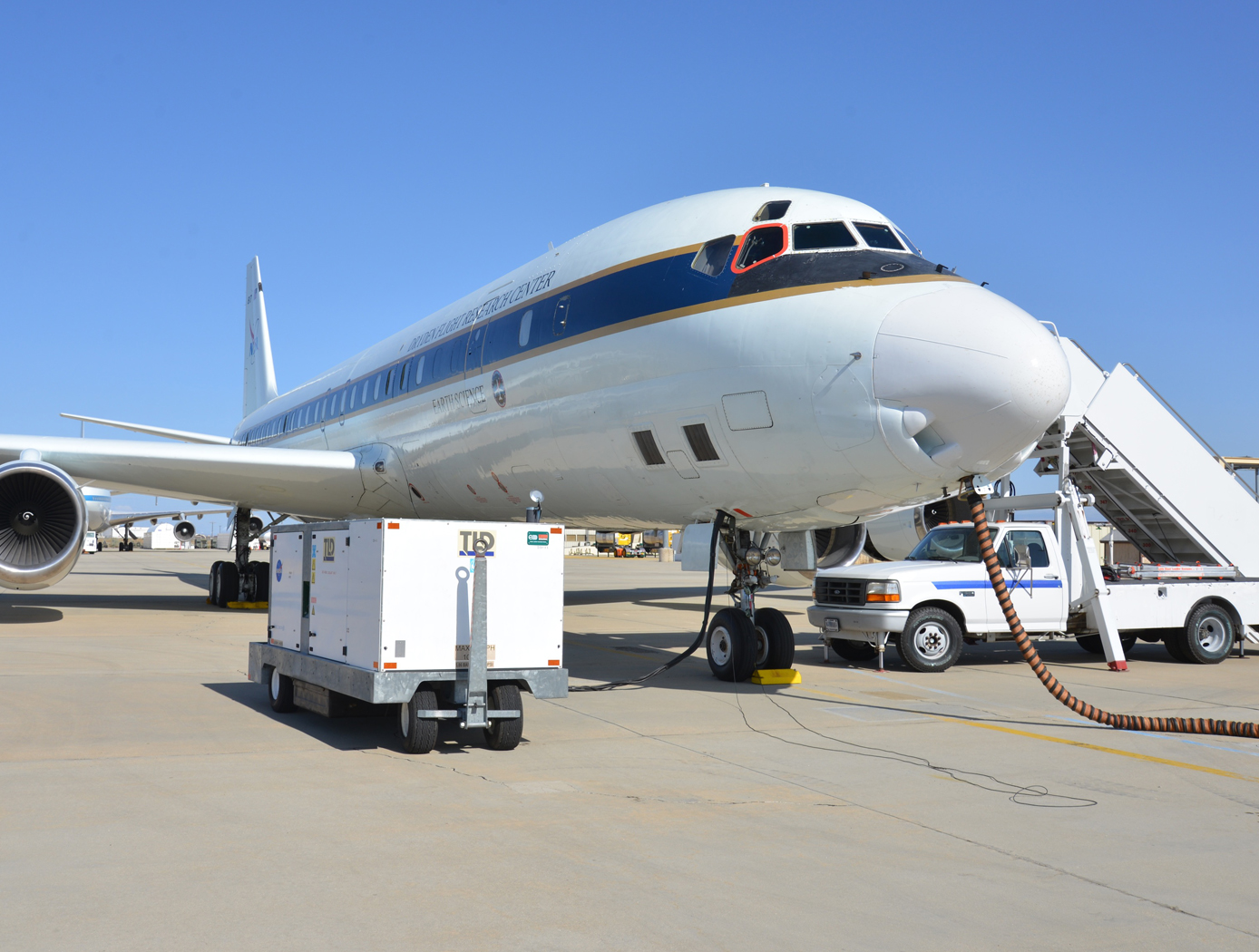
Highly Accurate Level Sensor
The FAA and Aircraft Industry recognize the need to reduce fuel tank explosion risk
by eliminating ignition sources and changing fuel tank design and maintenance.
This technology can be utilized to wirelessly sense the level of fuel in aircrafts, thus
mitigating risk of inadvertent electrical failures and sparks. NO wires enter the fuel
tank and the radio frequency transponder typically requires 10 milliwatts of power or
less.
The technology can be used for dielectric tanks, by simply applying the sensors to the
tank surface (as pictured). Through certain techniques the technology can be applied
on metal tanks with no wires entering the tank from the outside.
Currently, there are more than 20,910 jet aircraft in service. This presents a large
market opportunity for retrofitting this technology onto existing airplane fuel tanks
Rapidly evolving aviation services are expected to spur worldwide requirement for
36,770 new jet aircraft by 2033. This presents a growing market for new installations.